- 1Department of Agronomy, Kansas State University, Manhattan, KS, United States
- 2Department of Electrical & Computer Engineering, University of Illinois, Chicago, IL, United States
- 3Department of Plant and Soil Science, Texas Tech University, Lubbock, TX, United States
- 4Porous Media Research Lab, Department of Geology, Kansas State University, Manhattan, KS, United States
Sap flow measurement is one of the most effective methods for quantifying plant water use.A better understanding of sap flow dynamics can aid in more efficient water and crop management, particularly under unpredictable rainfall patterns and water scarcity resulting from climate change. In addition to detecting infected plants, sap flow measurement helps select plant species that could better cope with hotter and drier conditions. There exist multiple methods to measure sap flow including heat balance, dyes and radiolabeled tracers. Heat sensor-based techniques are the most popular and commercially available to study plant hydraulics, even though most of them are invasive and associated with multiple kinds of errors. Heat-based methods are prone to errors due to misalignment of probes and wounding, despite all the advances in this technology. Among existing methods for measuring sap flow, nuclear magnetic resonance (NMR) is an appropriate non-invasive approach. However, there are challenges associated with applications of NMR to measure sap flow in trees or field crops, such as producing homogeneous magnetic field, bulkiness and poor portable nature of the instruments, and operational complexity. Nonetheless, various advances have been recently made that allow the manufacture of portable NMR tools for measuring sap flow in plants. The basic concept of the portal NMR tool is based on an external magnetic field to measure the sap flow and hence advances in magnet types and magnet arrangements (e.g., C-type, U-type, and Halbach magnets) are critical components of NMR-based sap flow measuring tools. Developing a non-invasive, portable and inexpensive NMR tool that can be easily used under field conditions would significantly improve our ability to monitor vegetation responses to environmental change.
Introduction
Agricultural irrigation accounts for roughly 70% of global freshwater use and in some of the least developed countries, this number can reach upwards of 95% (FAO - SOLAW, https://www.fao.org/3/i7959e/i7959e.pdf). This rate of utilization is unsustainable and in many regions outpaces the natural restoration rate of the recharging aquifers causing a depletion in water resources (FAO – TAWAFSF, https://www.fao.org/home/en). Without improvements in plant water use efficiency or efficient water management practices, the rate of depletion would continue to rise to meet increased demand for agricultural products due to a combined increase in population and demand for global food supply (Hatfield and Prueger, 2015). In addition to these impending demands on the agriculture community, climate variability plays a more significant role in reducing production rates as extreme weather occurs more frequently and with greater intensity (FAO-CC14, https://www.fao.org/3/i5188e/I5188E.pdf). Recent studies focused on extreme events have shown that food production systems and water supplies are exceptionally vulnerable to climatic variability (FAO-CC14). More specifically, climatic variability is shown to negatively affect water availability and the ability to meet irrigation demands (Wada et al., 2013). The combination of increased population, demand for food and feed, and limited access to water would result in an increased demand for irrigation, which would ultimately deplete the natural groundwater reserves and threaten future agricultural productivity (Portmann et al., 2013; Meixner et al., 2016).
Limited water available scenarios would result in the decrease of amount of water in plants resulting in drought stress. Drought stress causes reduced cell size, photosynthesis, altered transcript abundances of transcription factor families, decreased turgor pressure and crop yields (Samarah, 2005; Kaya et al., 2006; Estrada-Campuzano et al., 2008; Jiao et al., 2021). Crop yield may decrease by 30% to 90% in fields under drought, although it varies from one crop species to another and depends on the intensity and the duration of stress (Hussain et al., 2019). Cereals are a significant part of our food supply, yet their agricultural production is heavily impacted by drought stress (Elliott et al., 2014; Kadam et al., 2014). The loss of agricultural yield caused by drought occurs in virtually every climatic region in the world (Daryanto et al., 2016). Since 1983, drought has reduced the overall yield of maize (Zea mays L.), rice (Oryza sativa L.), soybeans (Glycine max L.) and wheat (Triticum aestivum L.) in nearly 75 percent of all harvested areas (Kim et al., 2019). Similarly, using a modelling approach, studies have found that from 1980 to 2015 drought reduces yields of different crops like wheat, rice, soybean, maize by 21 - 28%, 25%, 21% and 39% respectively (Daryanto et al., 2016; Zhang et al., 2018).
Genetic background, environment, and management interact to determine crop yield and must be optimized to achieve the maximum yield potential. Recent studies have revealed that agriculture could expand northward due to the warming of high latitude regions and increasing food demand (King et al., 2018). Maize, oat (Avena sativa L.), and wheat cropping areas in Europe are likely to expand northward due to climatic warming (Elsgaard et al., 2012). Warming temperature in China has led to both westward and northward expansion of winter wheat (Yang et al., 2011) and maize (Hu et al., 2019). Due to the northward shift of the agricultural climate zone, many of the newly gained areas are linked to changes in climatic water balances. This is an important factor to consider in future land-use and management decisions (King et al., 2018). With a better understanding of plant water relations and soil moisture content, these agricultural land shifts can be accomplished more efficiently with more appropriate genotypic selections.
It is essential to understand mechanisms of plant water in response to soil and atmospheric drought. To effectively study plant water relations, the environmental and plant conditions must be accurately and rapidly measured and recorded. The study of this movement of water from soil to plant and to atmosphere is referred as soil-plant-atmosphere continuum (SPAC), which has become a dominant framework for understanding plant water movement (Deng et al., 2017). The most critical components of the SPAC are plant water potential, soil moisture content, plant uptake, evapotranspiration, and dynamics of xylem transport (Vicente et al., 2003; Fayer and Gee, 2004; Vidana Gamage et al., 2021). In plants, sap flow in the stem is used as an indicator of transpiration and water usage. Transpiration may be measured using the gas exchange system method. The rate of transpiration can be used to quantify the amount of water used by the plant and can lead to a greater understanding of plant water hydraulics under varying conditions (Steppe et al., 2015). Most popular techniques for measuring sap flow are based on heat balance and thermal dissipation. However, these are invasive techniques that are prone to multiple types of errors, such as misalignment of probes (Ren et al., 2017). Among technologies available to measure sap flow, nuclear magnetic resonance (NMR) is a non-invasive method, and several attempts have been made to manufacture portable and accurate NMR tools for sap flow measurement (Windt and Blümler, 2015).
Although the sap flow measurement literature is vast and extensive, it still lacks in a comprehensive review that addresses most recent advances and challenges. In this study, we particularly focus on nuclear magnetic resonance as a promising, non-destructive, and portable method for sap flow measurements. We overview mechanisms of transpiration and sap flow from biological viewpoint, address the importance of sap flow measurement in agriculture, review methods for quantifying sap flow and their advantages and limitations, and discuss portable nuclear magnetic resonance NMR system in measuring sap flow.
Plant hydraulics: The ascent of water
Plants have a complex transport system consisting of vascular bundles extending from roots to leaves and consisting of xylem and phloem (Figure 1). The most widely accepted approach for the ascent of sap is the transpiration cohesion-tension theory purposed by Dixon and Joly (1894). According to this theory, the upward movement of water is due to the negative pressure (suction) created by leaves during transpiration (driven by atmospheric pull) causing tension on the continuous water column in xylem (Dixon and Joly, 1894). Before entering the xylem, water is taken up by roots and must cross various cell layers, which act as a filtration system (Fiscus, 2015). Water travels through root epidermis and endodermis via nonexclusive pathways, such as the apoplastic, symplastic, and transcellular to reach xylem (Frensch and Hsiao, 1993). A network of non-living cells makes up the xylem tissue, which transports water from roots to leaves (Tyree and Zimmermann, 2002). In transpiration water passes through spongy mesophyll cells in leaves, it enters pores called stomata and through them into the atmosphere as water vapor (Figure 2).
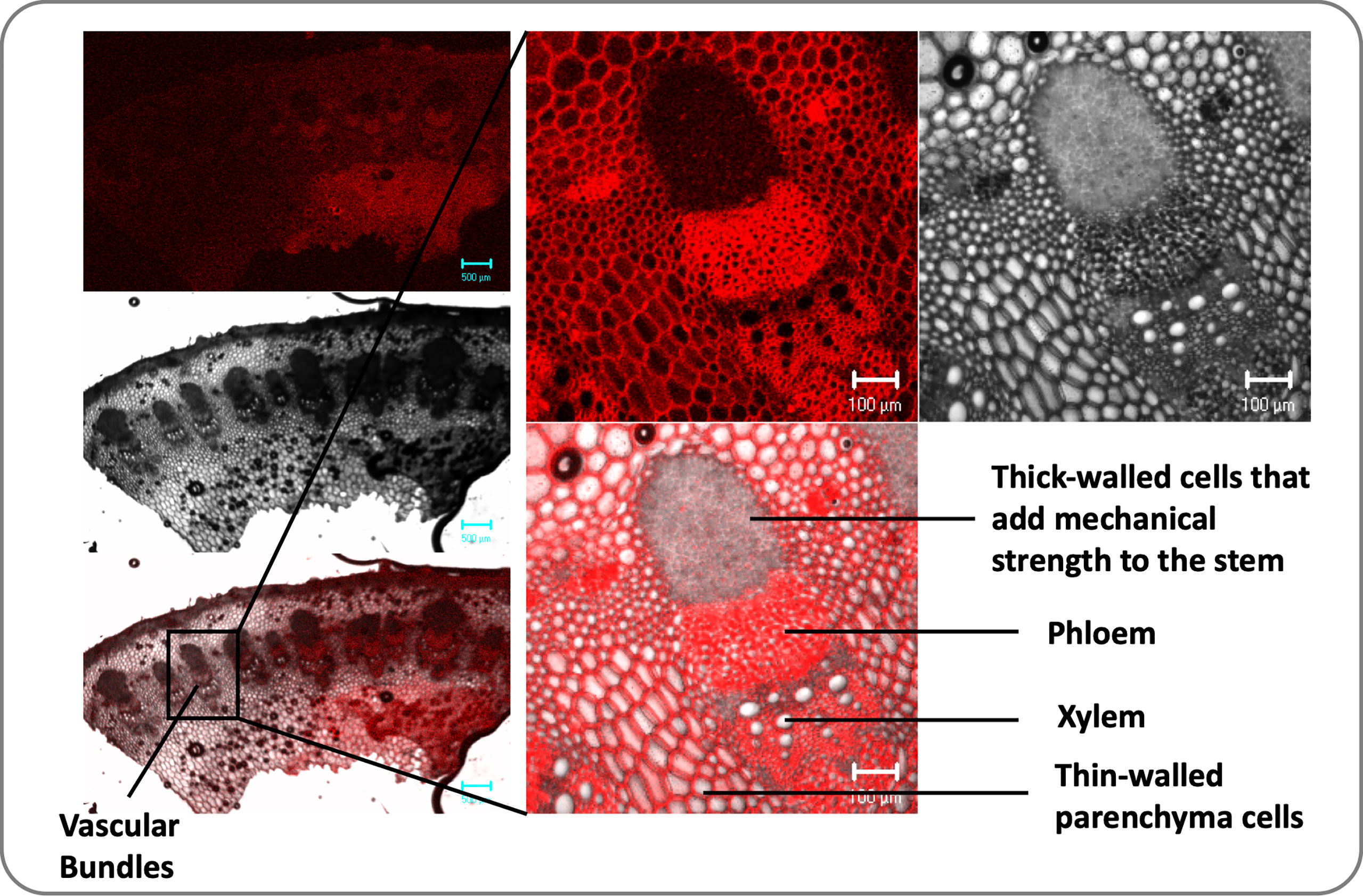
Figure 1 Transverse sections of the dicot stem (sunflower) illustrate vascular bundles’ arrangement. Vascular bundles are tubelike tissues that flow through plants, transporting different essential substances including water. Each vascular bundle consists of a one xylem and one phloem and there are several vascular bundles in the plant stem.
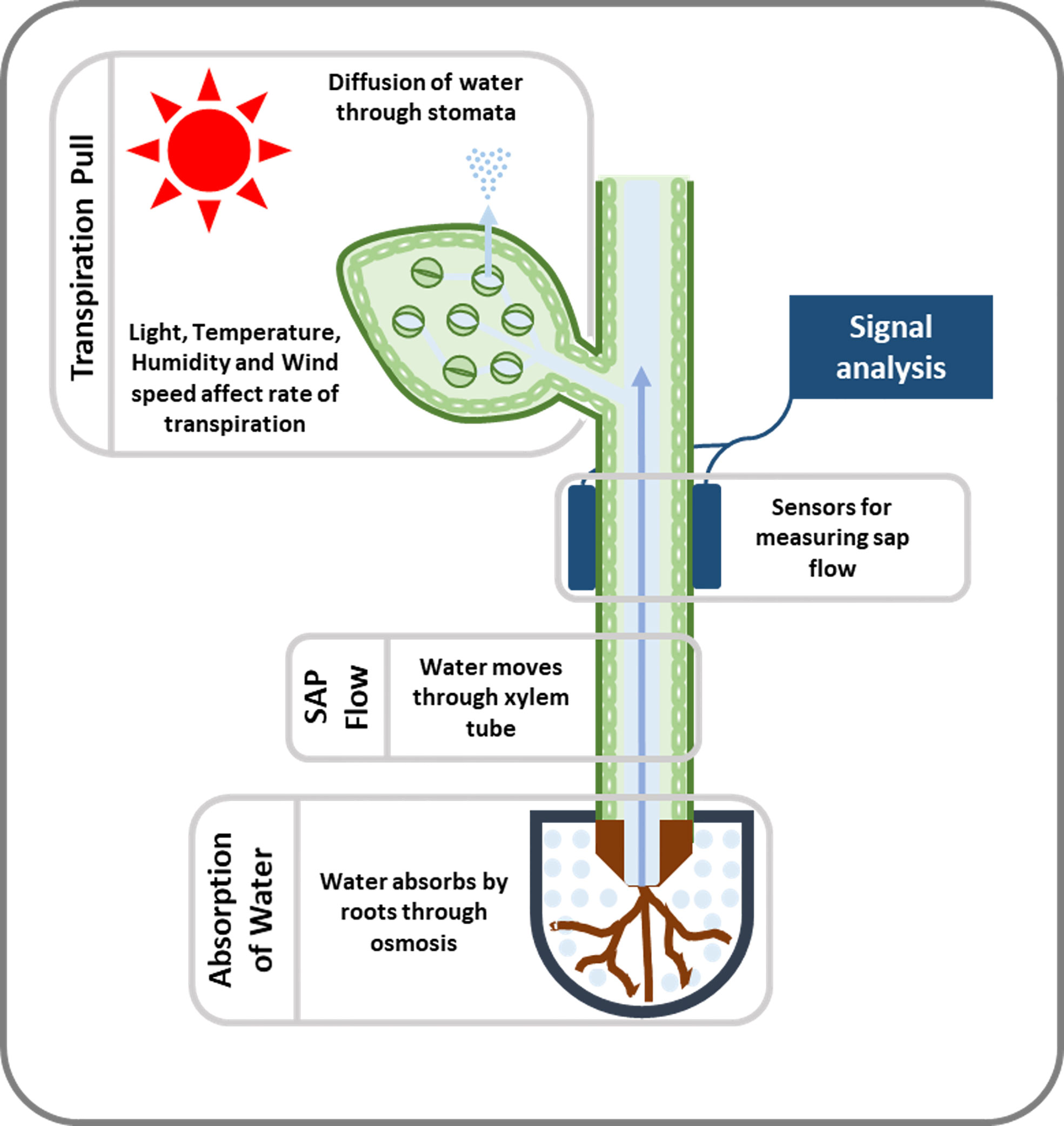
Figure 2 An illustration of the movement and measurement of sap flow. When leaves transpire, they generate negative pressure or suction that causes water to move upward. Sensing devices (used to measure sap flow) are attached to the stem of the plant or tree and detect this movement of SAP. To determine SAP velocity or SAP flux, the signal received from the sensors is detected and decoded.
In addition to transporting water, xylem sap carries inorganic nutrients from soil to other parts of a plant. Xylem sap contains sodium, calcium, potassium, magnesium, phosphorus, sulfur, boron, zinc, and copper (Pate et al., 1998; Jiang et al., 2004). Xylem sap also carries amino acids, proteins, plant hormones, and their metabolites (Bano et al., 1994; Bahrun et al., 2002; Kehr et al., 2005; Alvarez et al., 2006; Krishnan et al., 2011). However, the exact composition of xylem sap can vary significantly due to temporal, phenological, and environmental changes (Goodger et al., 2005; Alvarez et al., 2008; Krishnan et al., 2011).
Initially, it was believed that stomata closed at night and did not account for night-time water loss. However, several studies showed that many plants have significant night-time stomatal conductance, and 5-30% of water loss occurs due to night-time transpiration (Assaf and Zieslin, 1996; Oren et al., 2001). More specifically, Snyder et al. (2003) found that 11 of 17 different species of C3 and C4 plants used in their study showed night-time stomatal conductance. Positive effects of night-time transpiration include the ability to transfer nutrients to distal areas as well as prevent the accumulation of excessive leaf turgor pressure in the plants with high leaf osmotic potential (Donovan et al., 2001; Rohula et al., 2014).
Importance of sap flow measurement in trees and plants
Agricultural water management is one of the most important applications of sap flow measurement in a production environment. Irrigation management is critical in areas with insufficient water supplies and in drylands where water resource assessment and management are necessary for effective production as rainfall generally falls short of evapotranspiration demand (Koch and Missimer, 2016). The use of sap flow sensors helps irrigation management and timing as they provide accurate data on plants water demand (Jones, 2004; Esteves et al., 2015). Furthermore, such sensors are useful for managing irrigation based on soil and weather conditions. Based on sap flow measurement researchers have designed devices, which can calculate irrigation dose to replenish crop water needs (Fernández et al., 2008b; Fernández et al., 2008a; Ballester et al., 2013). Some of these devices can also be remotely controlled and provide daily irrigation amounts based on irrigation protocols (Fernández et al., 2008b).
Sap flow measurements for different species can provide insight regarding which one might be best suited under extreme conditions. For instance, by analyzing three Eucalyptus species, E. cladocalyx, E. melliodora and E. polybractea, it was found that E. cladocalyx tolerated saline soils, hotter and drier weather patterns more than the other two species (Doronila and Forster, 2015). In this study the heat ratio method was used to determine sap flow and tree water content. Measures of sap flow in these three eucalyptus trees (grown in the high saline condition) were made over 15 days including 3 days with temperature higher than 40°C. The mean ( ± S.D.) tree sap flux density measured per day was 287.8 cm3cm−2day−1 ( ± 87.2) for E. cladocalyx, 82.2 cm3cm−2day−1 ( ± 45.7) for E. melliodora and 81.5 cm3cm−2day−1 ( ± 42.5) for E. polybractea. A similar study was conducted on tropical tree saplings of Pterocarpus indicus, Lagerstroemia speciosa, and Swietenia macrophylla across wet and dry seasons in 2017–2018 by Andriyas et al. (2021), who estimated canopy conductance and transpiration from sap flux density over wet and dry seasons. They found that L. speciosa may perform better than S. macrophylla and P. indicus under future variable climatic conditions by balancing stomatal closure (Andriyas et al., 2021). In another study, (Suárez et al., 2021) examined diurnal patterns in sap flow from cacao trees grown in three types of agroforestry systems with differing levels of solar radiation. Based on the differences between cacao sap velocity, they developed a model to estimate transpiration and water use in cacao trees as well as to manage cacao agroforestry systems in the Amazon Rainforest. Further, in another study researchers showed the use of measuring sap flow in cocoa planting with the goal of increasing biodiversity should take into consideration the direction of land use and biodiversity transitions ( (Niether et al., 2020; Maney et al., 2022).
Another major application of sap flow measurement is to detect diseased plants (Deng et al., 2015; Roper et al., 2021). The vascular system is essential to plant health, and its damage can have an irreversible effect. Vascular occlusions, such as the formation of tyloses and gums, or the destruction of vessels by microbes, can disrupt hydraulic flux (Sun et al., 2013). Sap flow measurements were made on pine trees inoculated with Ophiostoma clavigerum i.e., a blue-stain fungus (Yamaoka et al., 1990). In this study heat pulse velocity (HPV) sensors were used to monitor sap flow daily for four weeks. The fungus (Ophiostoma clavigerum) was associated with mountain pine beetle (Dendroctonus ponderosae) and this pine beetle impaired tree sap flow (Amman and Schmitz, 1988) in lodgepole pine tree. A similar study revealed that sap flow in Norway spruce (Picea abies) ceased within 4–6 weeks after inoculation with a virulent fungus Ceratocystis polonica, an associate of the spruce bark beetle Ips typographus (Kirisits and Offenthaler, 2002). Sap flow measurements were used to detect reduced flux for tamarisk trees (Tamarix spp.) during defoliation by the salted cedar leaf beetle (Diorhabda carinulata) (Hultine et al., 2010). McElrone et al. (2010) showed that mature walnut trees affected by apoplexy disorder had hydraulic failure with the formation of tyloses in the Central Valley of California. More recently, a study by Ouadi et al. (2021) showed that sap flow dynamic, which is correlated with white-rot necrosis levels, is a useful indicator to predict plants suffering from Esca-grapevine disease.
In Canada and North America, xylem sap of several species within the genus Acer is extracted to produce maple syrup, a non-timber forest product of great value (Doner, 2003). Climate change has had a detrimental effect on maple syrup production (Matthews and Iverson, 2017). An analysis carried out at six different geographic sites in North America for over 2–6 years showed that sap collection advances by 4.3 days for every 1°C increase in March mean temperature (Rapp et al., 2019). Additionally, by 2100, the area of maximum sap flow is expected to shift northward by 400 km (Rapp et al., 2019). In that study, sap flow measurements were used as an important tool in revealing that the changing climate conditions affected the number of freeze/thaw cycles, sap collection days, and total sap amount.
Techniques used for quantifying sap flow
To determine the amount of sap flowing through a surface per unit time, proposed methods measure either flow rate (gh-1) or flux density (cm3cm-2h-1) (Vandegehuchte and Steppe, 2012). Different approaches available to measuring sap flow are based on thermodynamics, electrodynamics, or magneto-dynamics concepts. Of the available methods, those based on thermodynamics are among the most widely used (Čermák et al., 2004). Many thermodynamic-based sap flow sensors are available commercially. Thermodynamic techniques for sap flow measurement can be categorized into three major groups: heat balance (HB), thermal dissipation (TD), and heat pulse velocity (HPV) (Table 1). The HB method involves circling stem with a thin flexible heater and surrounding it with foam insulation allowing a known amount of heat to be applied (Sakuratani, 1981). This approach considers both conduction and convection losses in stem, heat loss due to the mass flow of water in stem are estimated (Sakuratani and Abe, 1985). In the TD method, two needles mounted with a thermocouple are inserted radially in the tree stem 10cm apart to detect convective heat transfer (heat transported with the sap stream). In the HPV method, a pulse of heat is injected into xylem, and its passage is detected further along the flow direction. By measuring the pulse travel time, the flow velocity is estimated (Forster, 2017). These heat-based methods are majorly used for sap flow measurement more than any other methods. The advantage of using these heat-based methods for sap flow measurement is that they are comparatively easy to use than other methods, portable, and most of them are commercially available. But there are many major drawbacks associated with these methods. The heat-based methods involve inserting probes, which causes wounding and mechanical damage to the plants. Misalignment of probes may also result in substantial uncertainties in sap flow measurements. For example, the study by Burgess et al. (2001) demonstrated that a 1-mm difference in probe spacing in the compensation heat pulse method (CHPM) would render 20% error in the heat pulse velocity. In another study on Eucalptus marginata, Bleby et al. (2004) found a 2-mm error in spacing caused a 100% error in sap velocity determination. Moreover, installing these components (like probes) leads to the species-specific wounding responses, resulting in accounted and unaccounted errors in measurements (Bush et al., 2010; Wullschleger et al., 2011). In plants, a wound due to a drill hole generally induces tyloses formations (Barnett, 2004) that cause significant reductions in hydraulic conductance and sap flow (Sun et al., 2006). Heat pulse methods use a default value of thermal diffusivity (heat tra\nsfer rate). However, evidence show that the value of thermal diffusivity changes at a faster rate than the period of measurement resulting in an overestimation of sap flow (Looker et al., 2016). Other parameters, such as stem moisture content and sapwood radial variability, can also contribute to errors during sap flow measurement and produce unreliable results. A comparative study showed that the actual flux density was underestimated by 35, 46, and 60% using the heat pulse velocity, heat field deformation, and thermal dissipation respectively (Steppe et al., 2010).
To reduce errors in sap flux density measurements, one may use a single probe containing three-temperature sensors that can autocorrect misalignment (Ren et al., 2017). With probe spacing corrections applied in this study, the root mean squared error (RMSE) values for CHP, HR, and T-max methods decreased from 4.82, 3.09, and 2.73, respectively, to 3.1, 2.18, and 0.82 (Ren et al., 2017). In addition, a sap flow sensor design with all sensor components on a single integrated circuit board helps minimizing misalignment errors (Jones et al., 2020). There are still other factors needed to be eliminated in future research, such as differences in axial versus tangential thermal properties, distance-dependent thermal properties of trees, and wounds that may cause errors in sap flow velocity.
In the literature, several non-invasive techniques to measure sap flow have been proposed. Helfter et al. (2007) used a non-invasive pulsed laser-based technique to monitor xylem and phloem flow in woody plants. In their method, infrared lasers produced heat pulses were used to detect sap flow. The laser source produces heat on the stem surface, and an infrared camera monitors the heat propagation externally, and finally using thermometric data heat pulse velocity is calculated. Another non-invasive way is measuring sap flow velocity using different dyes e.g., fuchsin, safranin, and eosin. These are one of the oldest methods to visualize water-conducting pathways. Xylem specific dyes like acid fuchsin and crystal violet were used to study conductive area and flow pathways from roots to shoots of 20-year-old Thompson Seedless and 8-year-old Chardonnay grapevines (Harris, 1961; McElrone et al., 2021). Using different dyes sapwood of various conifers was studied including Pseudotsuga taxifolia (Poir.) Britt., Pinus nigra Arnold, and P. radiata D. Don. Acid fuchsin uniformly stained the late wood because it diffused easily, but safranin did not, and it remained uncolored (Harris, 1961). Acid fuchsin dye is also used to study patterns of water movement in four species of gymnosperms and seven of angiosperms (Kozlowski and Winget, 1963). Likewise, eosin dye helps measure the conducting capacity of lateral veins of the wheat leaf (Altus et al., 1985). A major disadvantage of using dyes is that it is not known whether they would travel as far as the sap flow before they deposit on the vessel walls (Marshall, 1958). Furthermore, dyes would never indicate variation in speed along a dyed path but only show the maximum speed along such a path (Marshall, 1958).
Researchers have used a variety of methods based on heat transport (Marshall, 1958), nuclear magnetic resonance microimaging (Köckenberger et al., 1997), magnetic resonance imaging (Homan et al., 2007), optical techniques (Helfter et al., 2007), particle-traced X-ray imaging (Ahn et al., 2013), acoustic emission technique (Zweifel and Zeugin, 2008), and high-resolution computed tomography (Brodersen et al., 2019) to determine sap flow in plants (Alarcón et al., 2000). Lee and Kim (2008) explored plant water-refilling processes using synchrotron X-ray microimaging. Then, Kim and Lee (2010) used non-destructive synchrotron X-ray imaging to monitor sap flow through the xylem vessels of rice leaves. Positron emission tomography (PET) scanners is advancing as a powerful functional imaging technique to decipher in vivo the function of xylem water flow (with 15O or 18F), phloem sugar flow (with 11C or 18F) (Hubeau and Steppe, 2015). In a recent study, plant PET scanners were used to study phloem vulnerability under hotter drought conditions in Populus tremula (Hubeau and Steppe, 2015). A magnetic resonance imaging (MRI) scan of a living plant stem can also be used to determine the location of the xylem vessels and understand their status. Using this approach, if these vessels were filled with liquid, the velocity, and direction of the movement of this liquid, and metabolites present in it can be identified (Windt et al., 2009; van As et al., 2009).
Magnetic resonance imaging (MRI) is most probably the only non-invasive techniques used to measure both xylem and phloem sap flow. However, the currently available equipment are bulky, expensive, and not suited for field measurements. Instead, various intrusive techniques use heat to trace sap flow in the field. Due to probe implantation, these methods disrupt the flow being measured and are unsuitable for phloem monitoring due to the fragility of the tissue.
Nuclear magnetic resonance for sap flow measurements
Nuclear magnetic resonance (NMR) is a phenomenon when a strong magnetic field and the nucleus-based phenomenon is basically related to the absorption and re-emission of electromagnetic wave radiation. This phenomenon was introduced in 1938 (Rabi et al., 1938; Rabi et al., 1939). In the early 1940s, Felix Bloch and Edward Purcell independently developed the NMR method for measuring nuclear magnetic moments, for which they shared the Nobel Prize in 1952. NMR applications developed rapidly over the next 50 years. Nuclei with odd numbers of protons and neutrons have non-zero spins, making them act as tiny bar magnets. Their orientation is normally random and there is no net magnetic field, so they cannot generate an NMR signal. In NMR, when these nuclei are subjected to an external strong and homogenous magnetic field, nuclei spins are orientated along the magnetic field direction; some in parallel (low energy) and some others in antiparallel directions (high energy). This results in a net magnetic moment generated which is aligned with the external magnetic field. This net magnetization can be pushed away from the alignment using a second magnetic field applied perpendicular to the external magnetic field with an excitation coil. Net magnetization returns to the external magnetic field when this second field is turned off, generating a radiofrequency signal which is detected at the detector coil. This signal forms the basis of NMR imaging and spectroscopy.
The energy difference between the two states of nuclei spin under an external strong magnetic field is very small and corresponds to a range of frequency of the EM spectrum. A short duration radio frequency signal is used to flip the nuclear spin from the spin-aligned state of low energy to the spin-opposed state of high energy. Thereafter, the charged nucleus will exhibit processional motion with a specific frequency, known as the Larmor frequency. The actual magnetic field created at a nucleus is also dependent on the electrons surrounding it, which creates an ionic environment around it which leads to a variation in the frequency of resonating particles, known as chemical shift. This change magnetic field is known as shielding. Energy is absorbed by the nuclei during the transition from lower to higher energy levels and released during the opposite process. The process of returning to the original energy state is known as relaxation. The two most common types of relaxation are spin lattice relaxation (T1) and spin relaxation (T2). T1 relaxation, also known as spin lattice or longitudinal relaxation is the time constant used to describe when ~63% of the magnetization has recovered to equilibrium. T2 relaxation involves energy transfer between interacting spins via dipole and exchange interactions. Spin-spin relaxation energy is transferred to a neighboring nucleus. Now there are two NMR methods continuous and pulsed method. In continuous wave NMR method, a sample in a static magnetic field is applied by RF perpendicular to the sample and when an excitation frequency is reached, oscillation is observed at the detector. In the pulsed case, one applies a sequence of RF pulses called π-pulses (180-degree pulses) or π/2-pulses (90-degree pulses) and looks for “free induction decay” and “spin echoes”. Use of Pulse NMR experiments are most popular these days.
Capturing all details of these reaction can be conducted by accurate measurement systems. The captured waveforms which are known as NMR signals, release fundamental features regarding the understudy materials; see Figure 3 (Callaghan et al., 1991). Concept of NMR has been applied in many research areas e.g., in plant studies to determine moisture in apple (Shaw and Elsken, 1956), unfrozen water in winter cereals at subfreezing temperatures (Gusta et al., 1975), and xylem embolisms in pine wilt disease (Umebayashi et al., 2011). One advantage of NMR is that it can spatially resolve both static and dynamic parameters and generate data in a non-destructive manner from the interior of a sample.
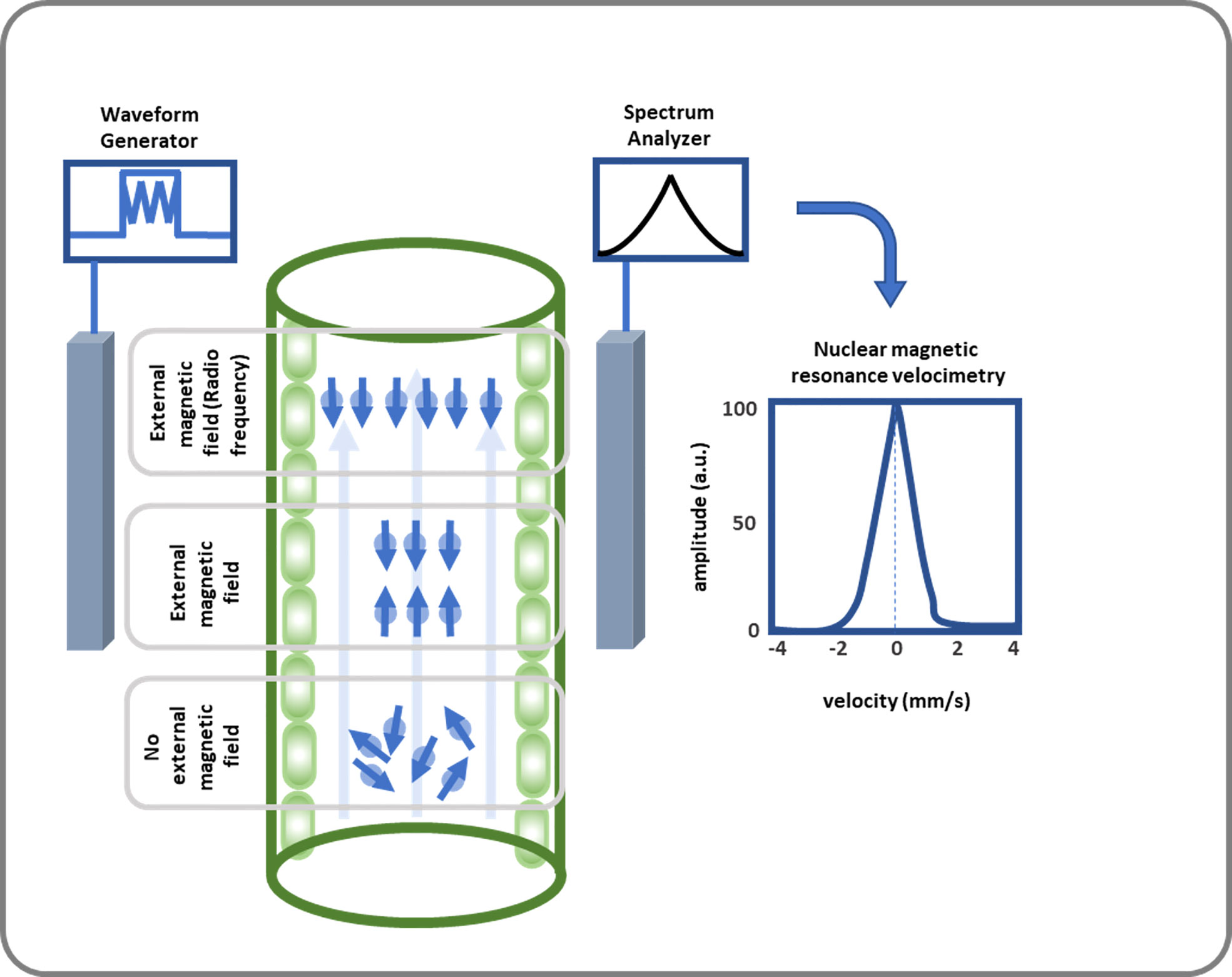
Figure 3 This diagram illustrates how SAP flow is measured using NMR. SAP flow measurement using NMR includes a strong magnetic field where 1H nucleus gains a quantum mechanical property callspin at. Then an NMR signal is created by applying RF energy equal to the Larmor frequency, which leads to nuclei transitioning from their groan und to excited state, and spins being oriented oppositely or antiparallelly. After receiving, amplifying, and further analyzing the signal, the volumetric flow rate of the xylem and phloem is calculated.
NMR can be used in different applications where non-destructive measurements are required. NMR imaging is also widely used to measure sap flow (Table 2). van As and Schaafsma (1984) investigated water flow through the stem of an intact cucumber plant using pulsed NMR. In their study, the NMR signal due to high stationary water was cancelled out so that it doesn’t interfere in the detection of flowing water. This made it an important application for measuring water flow to plants with high stationary water content. Using NMR microimaging, Köckenberger et al. (1997) measured the phloem and xylem water flow in castor bean seedlings. This study demonstrated for the first time that water is internally circulated between phloem and xylem. Even in absence of significant transpiration or evaporation, there is water maintenance through this water circulation. Fast gradient echo sequence (FLASH) imaging of flow velocities, as a fast-imaging NMR method, was introduced by Rokitta et al. (1999). Their method achieved a six-fold reduction in imaging time while maintaining a similar signal-to-noise ratio to previous flow NMR imaging techniques. Scheenen et al. (2000) developed the pulsed field gradient (PFG) NMR technique combined with turbo spin-echo imaging, which resulted in an accurate and spatially resolved sap flow measurement. Windt et al. (2006) used NMR imaging for diurnal dynamics study of phloem and xylem transport in poplar (Populus spp), castor bean (Ricinus communis), tomato (Solanum lycopersicum) and tobacco (Nicotiana tabacum). They found large diurnal variation in xylem flux and small or no change in phloem flux.
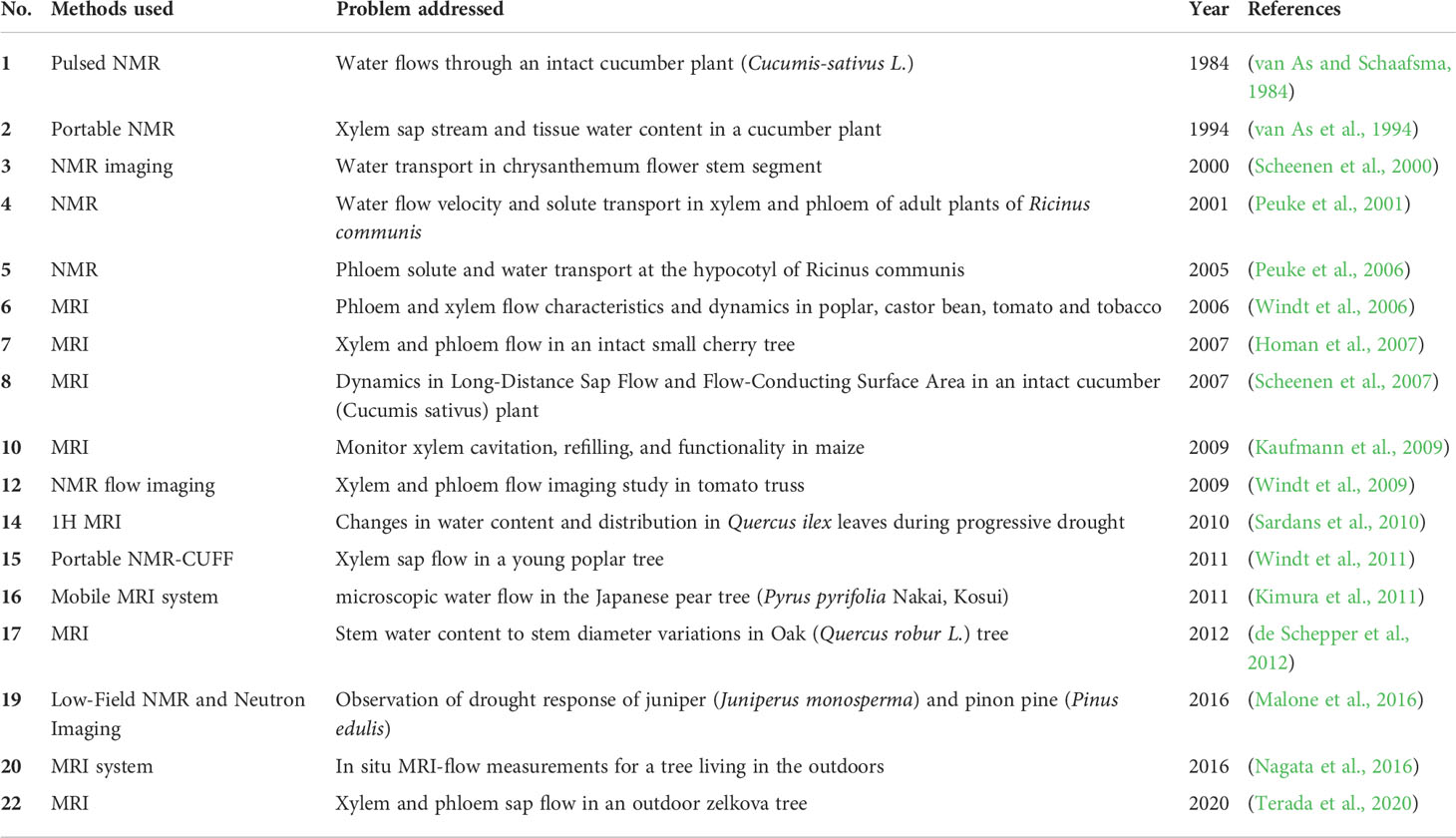
Table 2 Different studies used NMR and other imaging methods to study sap flow measurement in plants and trees.
Using NMR flow imaging, Windt et al. (2009) demonstrated that, in a developing tomato truss, 75% of net influx into a fruit occurred through the xylem and the remaining 25% via the perimedullary region, which includes both phloem and xylem. Using NMR methodology, the moisture content in wood samples could be instantly determined from the mass and amplitude of free-induction-decay (FID) signals and found this methodology to be more precise and reliable than the gravimetric methods regardless of wood species (Merela et al., 2009). Furthermore, NMR imaging can determine the level of hydraulic conductivity in detached stems. Using MRI, de Schepper et al. (2012) showed that elastic bark tissues contribute most to the daily depletion of internal stem water storage. In another study, low field NMR and neutron imaging were used to deduce aspen tree (Populus tremuloides) drought response (Malone et al., 2016). NMR imaging was also used for grape (Vitis vinifera) stems to show that basal leaves were more prone to embolism than apical leaves and these leaves shed to prevent water loss and protect the hydraulic integrity of the plant (Hochberg et al., 2017).
The variety of possible applications of NMR makes it an extremely useful tool to understand plant water relations. However, experiments and applications are currently limited to solely lab-based measurements. To take full advantage of the potential applications of NMR, the technology must be advanced and integrated into a portable system which can be taken to the field condition. In the following, we address current challenges with designing a portable NMR tool for sap flow measurements in plants.
Challenges in designing portable NMR for sap flow measurement
NMR demonstrates promising results as a tool for sap flow measurement (Windt et al., 2011). A typical NMR spectrometer system consists of the magnets (to generate an external magnetic field), lock (used to control magnetic field in the sample so that the resonance frequencies do not drift), shim coils (to adjust the homogeneity of a magnetic field), gradient coils (used to produce deliberate variations in the main magnetic field B0), RF coils (to generate the RF magnetic field that excites the nuclear spins, and to detect the MR signal), and a spectrometer (a receiver which detects the magnetic resonance signal) (Figure 4i). NMR imaging system can measure the presence and movement of water quantitatively and non-invasively. However, many restraints like complexity and bulkiness in the NMR instrument limit its applicability to field studies. There is a major limitation with NMR in plant sciences due to the lack of small-scale and portable NMR tool that can be used in greenhouses and field conditions. Moreover, these methods generally involve complicated procedures and data processing techniques (Windt et al., 2021; Meixner et al., 2021).
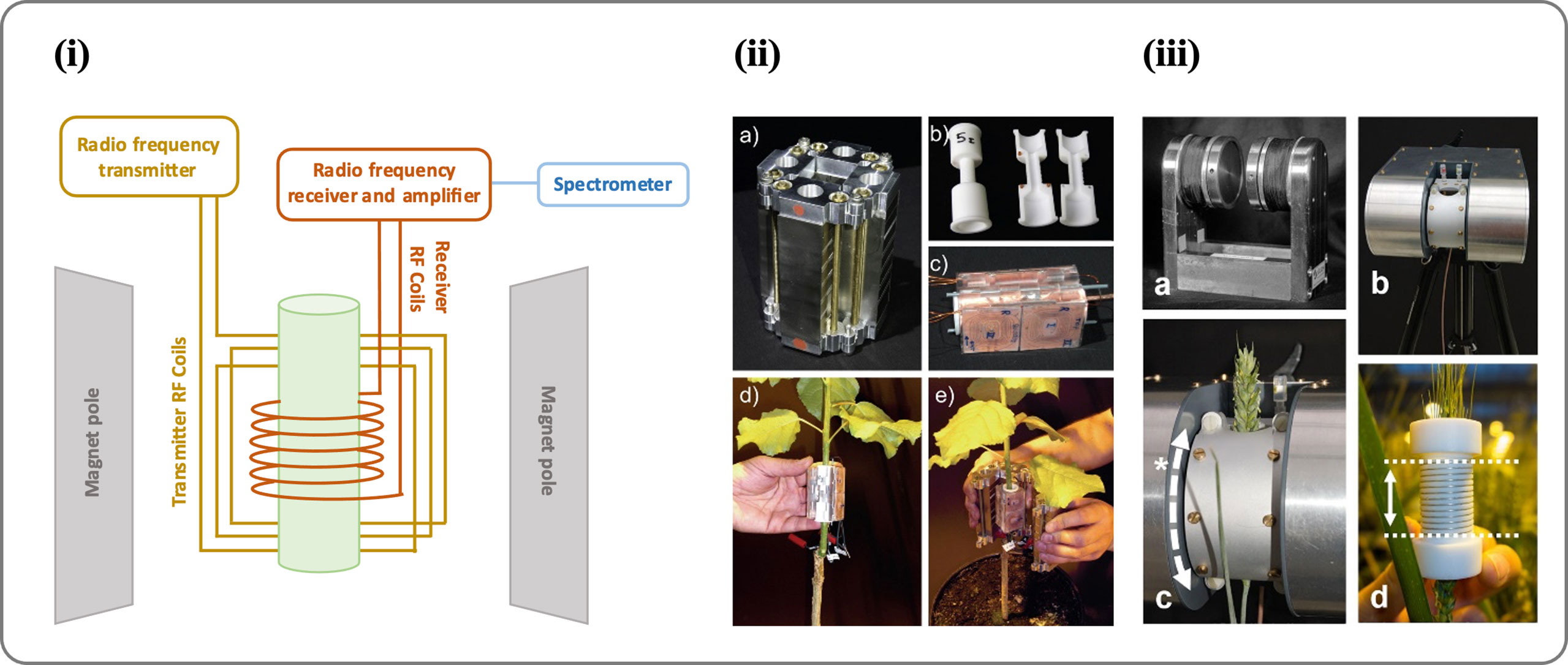
Figure 4 (i) An image showing the different parts of an NMR spectrometer. (ii) Portable NMR CUFF magnet system (source: Windt et al., 2011). An NMR-CUFF prototype, consisting of a Halbach magnet, is shown in this image; (A) shown is the template for an RF-coil; (B) the hinged, plane-parallax gradient system assembly with an RF-coil inside; (C) mounting the RF coils; and (D) enclosing the plant stem with the NMR-CUFF. (iii) Mobile NMR Mouse sensor made from a C-shaped magnet (source: Windt et al., 2021). An image of the magnet (A) shows a permanent C-shaped magnet, (B) an insulating housing or adjustable stand, (C) a probe housing that can be rotated around the magnet poles (*) to the sample angle, and (D) solenoidal RF coils with dotted lines indicating their sensitive volume (25 mm). For more details see Windt et al., 2021.
Homogeneity and strength of magnetic field
According to the main purposes of the NMR technology, providing a strong and homogenous static magnetic field is essential to have a better observation of the transition in nuclear spin. It is worth mentioning that there is a direct correlation between the Larmor frequency, the spin bearing nucleus and the static magnetic field strength. Also, by intensifying the constant magnetic field in the test region, contrast to noise ratio could be improved considerably (Okada et al., 2005). Halbach arrays provide a homogenous and strong magnetic field (Raich and Blümler, 2004), a significant characteristic for NMR-based imaging and velocimetry applications. This is due to a uniform flux that is generated based on the orientation of segmented magnets in the Halbach arrays (Halbach, 1980). In other magnet structures, such as north-south magnets, extra iron plates are commonly needed to be added on exterior face of the ring to minimize the leakage flux and have stronger magnetic field within the ring. The challenge here will be resulting less homogenous magnetic field due to the impact of iron plate of uniformity of flux. In Halbach magnet structures, however, this extra iron plate is not required due to the orientation of segmented magnets, which results in negligible leakage flux. This makes the Halbach magnet-based tool lighter with more homogenous magnetic field within the ring compared to other magnet types. However, designing NMR with Halbach arrays has some challenges as well. For instance, multi-direction pulling forces may be strong enough that an openable mechanical structure could not be released easily during a test cycle. Thus, it is essential to provide an appropriate design to lessen consequences of the existing pulling force in the NMR (Li et al., 2021). The mechanical structure should be in a way that the Halbach rings can be opened with minimal forces required, which can be simulated using finite element analysis tools. It should be mentioned that the excessive extent of pulling force can progressively misalign the finalized NMR mechanical structure, and such a misalignment can demote the level of magnetic field homogeneity (Koch et al., 2009).
Moreover, error in the production process of magnetic parts is another challenge for NMR design. This issue has some adverse effects on the homogeneity of magnetic fields which can be observed by the electromagnetic studies in a finite element analysis tool. To develop an optimal, compact, and portable NMR tool, the magnetic pulling force must be optimized by considering the acceptable magnetic strength. For a comprehensive study regarding the force on a magnetic dipole and differences in pulling forces that are experienced by dipoles see Boyer (1998). Another main challenge to develop a portable NMR device is the homogeneity of magnetic arrays. The magnetic field homogeneity would be at the highest level when the mechanical structure of the NMR has an infinite length. However, in real conditions having a lengthy instrument is not practically feasible. In addition, as stated earlier, inaccuracy in the manufacturing process can effectively decrease the homogeneity. To overcome this challenge, magnetic arrangement for novel discrete Halbach layout approach is used in numerous designs processes (Soltner and Blümler, 2010). Thus, the final magnetic structure with limited length can have a homogenous field by placing small permanent magnets in an optimal location, which are found by running an optimization algorithm that mostly relies on analytical equations (Huber et al., 2018). Moreover, the mechanical strength of these structures should be considered in the fabricating process. When the number of the magnetic segments increases, the number of points that are vulnerable to be separated are increased as well. This is due to multi-direction pulling forces that are mostly effective between these Halbach magnets. This condition becomes more crucial when the NMR becomes more compact. It should be mentioned that by decreasing the number of magnetic parts in the structure, the mechanical strength of the design is upgraded noticeably at the cost of less homogenous magnetic field.
Optimum dimensions of the test region
Determining the optimum dimension for the region of the test is another challenge in portable NMR devices (Klein et al., 2021). The air gap length can alter the homogeneity level of the magnetic field in the test region. Decreasing the airgap in the test region would improve the homogeneity level. However, this can constrain the practical development of the NMR tool from two points of view: first, the pulling force is increased drastically, and second, there would not be enough effective space for doing specific tests which need a holder around the understudy stem.
Imaging and velocimetry challenges
Regarding the imaging and velocimetry applications in NMR devices, having a well-designed radio frequency (RF) coil is important to apply effective exciting signals on the understudy sample. Furthermore, in some NMR tools a receiving coil needs to be designed with high accuracy to observe the transition in nuclear spin. Generally, a static field is generated by a mechanical magnetic module, and if this structure is openable, a misalignment may occur in the structure by repeating the test, which is mainly because of the high amount of pulling forces. Thus, designing a robust openable structure to have an accurate imaging is crucial.
RF generator, amplifier, and spectrometers are required devices used to imaging and measuring velocity. The level of accuracy for generating pulses and receiving transitions in nuclear spin have significant impact. If the exciting signals have some deviations from their defined positions or the signal quality cannot not satisfy the minimum imaging constraints, the signal to noise ratio might have a lower value. Noted that, due to the high sensitivity of the high-frequency transmitter and receiver coils to the surrounding area, it is recommended that the test is carried out under conditions where external interfering disturbances are minimal. Certainly, satisfying these constraints to obtain a high-resolution image has numerous electromagnetic challenges. Addressing such constraints enables realization of an effective portable NMR tool for sap flow measurement. Despite such challenges, some research groups have succeeded in making compact and portable NMR tool so that experiments can be conducted in situ(climate chambers, greenhouses, or the natural environment) rather than transporting plants to the lab (Musse et al., 2017). In 1998, a portable NMR device named NMR-MOUSE (Figure 4iii) was constructed by Blümich et al. (1998) and used for a variety of applications, such as analysis of porosity of the materials (Blümich et al., 2010), stratigraphy of paintings in a non-destructive and non-invasive manner (di Tullio et al., 2016), leaf water status (Capitani et al., 2009), and moisture fraction in wood (Casieri et al., 2004). In a recent study, NMR-MOUSE was used to analyze the circadian variation of root water status in three herbaceous species (Nuixe et al., 2021). A further step in developing portable NMR is the use of permanent C-shape magnets (Rokitta et al., 2000). This type of magnet has good homogeneity and field strength, although it depends on the field strength and air gap. Nonetheless, its heavy weight limits its usefulness as a portable NMR device. Another study developed a Halbach-type permanent magnet arrangement (Figure 4ii) that could be opened from one side and closed around a target object: the NMRCUFF (for Cut open, Uniform, Force Free) (Windt et al., 2011; Blümler, 2016). Further this NMR CUFF based portable NMR was used to measure xylem sap flow in a poplar tree (Populus nigra) (Windt and Blümler, 2015). However, it is still a long way off from taking this portable NMR technology to the field and measuring sap flow in real-time. One of the recent attempts is made by Windt et al. (2021) in which all components are mounted including the magnet and spectrometer (with a total weight of 45kg) on a handled trolley and transported to the field. An imaging system weighing 45kg was used to measure quantitative water content in apple orchards (Meixner et al., 2021).
Concluding remarks
Compact and portable NMR can be a potential solution as a powerful tool for gauging water movement in plants non-invasively. A non-invasive, portable, inexpensive NMR methodology for monitoring changes in plant water status that can be used in field conditions would greatly improve the understanding of vegetation responses and environmental feedback. Monitoring sap flow continuously at short time intervals is possible using NMR, which can help manage irrigation water. In future, new remote sensing tools and products with portable NMR can provide the best opportunities for measuring and modelling ecosystem-scale water fluxes (Figure 5). Moreover, there is a need for a global database where individual datasets containing sap flow data provided by contributors worldwide can be stored, analyzed, and used to develop prediction models based on which different models based on sustainable agriculture plants and water availability can be predicted which can be helpful in future changing climate condition.
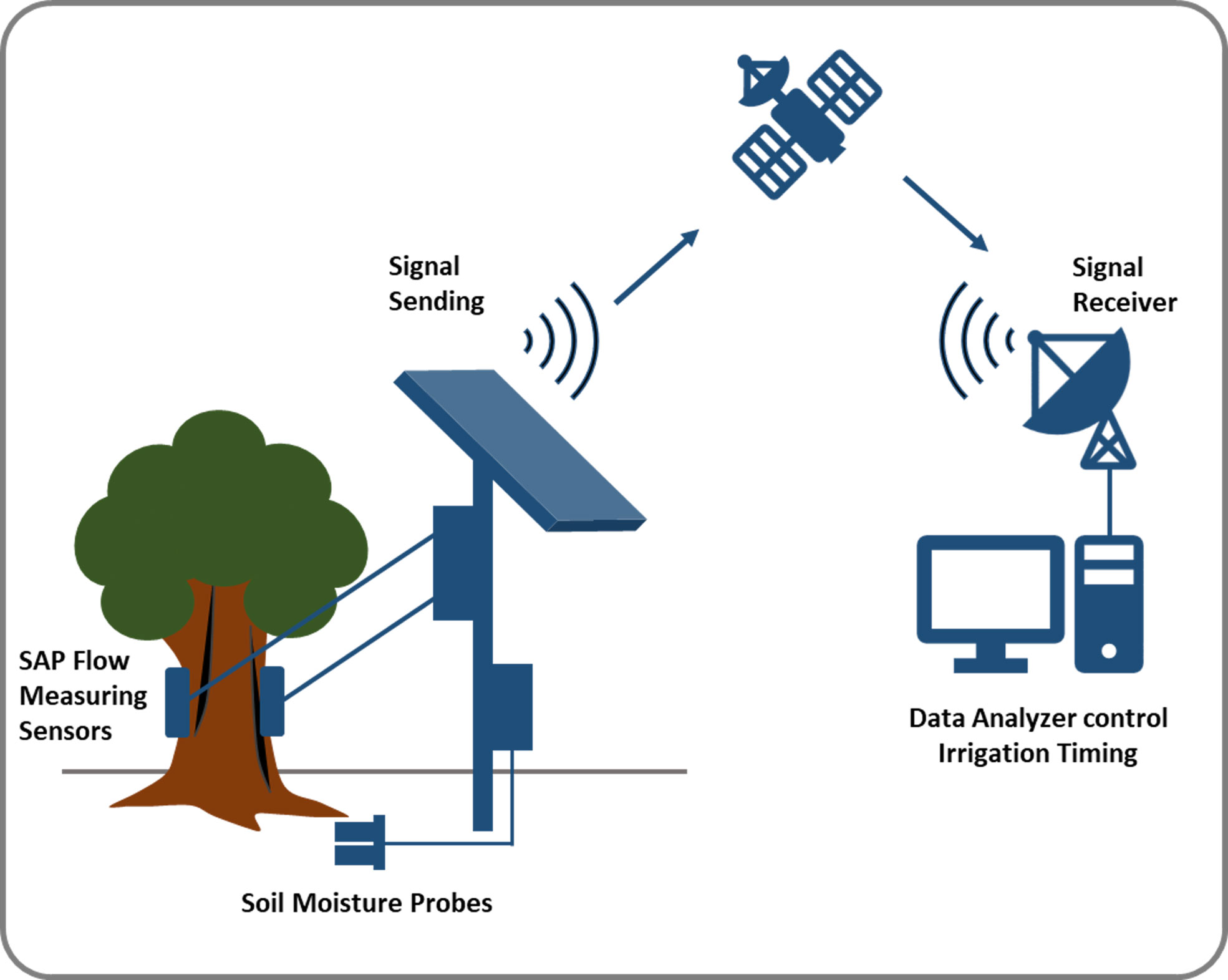
Figure 5 Model for monitoring the water content of plants using NMR combined with remote sensing tools for irrigation time management. In, future we need a portable NMR which can be used in the field and can be remotely controlled and remotely receive the data for the analysis.
Considering future climate change, the availability of an NMR sensor is critical for plant health and irrigation management. It is still challenging to measure sap flow using NMR outside a strictly controlled environment. However, with the intense research going on in developing portable NMR, in the near future we expect to use this technology to monitor sap flow in plants and trees non-invasively in the field.
Author contributions
The first draft of the manuscript was written by RK, MH, and NH. MS, SJ, and BG revised the manuscript. All authors read and approved the final manuscript. All authors contributed to the article and approved the submitted version.
Funding
This research was supported by the US National Science Foundation (NSF) through the Plant Genome Research Program (PGRP). The authors acknowledge the NSF for financial support through grant no. 1936376.
Acknowledgments
The authors are grateful to Gerard Kluitenberg, Kansas State University, and Carel Windt, Forschungszentrum Jülich, for insightful discussions.
Conflict of interest
The authors declare that the research was conducted in the absence of any commercial or financial relationships that could be construed as a potential conflict of interest.
Publisher’s note
All claims expressed in this article are solely those of the authors and do not necessarily represent those of their affiliated organizations, or those of the publisher, the editors and the reviewers. Any product that may be evaluated in this article, or claim that may be made by its manufacturer, is not guaranteed or endorsed by the publisher.
Abbreviations
CHPM, Compensation heat pulse; FAO, Food and agriculture organization; HFD, Heat field deformation; HPV, Heat pulse velocity; HR,Heat ratio; MRI, Magnetic resonance imaging; NMR, Nuclear magnetic resonance; SHB, Stem heat balance; TD, Thermal dissipation; TSHB, Trunk sector heat balance; TTD, Transient thermal dissipation.
References
Ahn, S., Jung, S. Y., Lee, S. J. (2013). Gold nanoparticle contrast agents in advanced X-ray imaging technologies. Molecules 18, 5858–5890. doi: 10.3390/molecules18055858
Alarcón, J. J., Domingo, R., Green, S. R., Sánchez-Blanco, M. J., Rodríguez, P., Torrecillas, A. (2000). Sap flow as an indicator of transpiration and the water status of young apricot trees. Plant Soil 227, 77–85. doi: 10.1023/A:1026520111166
Altus, D. P., Canny, M. J., Blackmann, D. R. (1985). Water pathways in wheat leaves. II. water-conducting capacities and vessel diameters of different vein types, and the behaviour of the integrated vein network. Funct. Plant Biol. 12, 183–199. doi: 10.1071/PP9850183
Alvarez, S., Goodger, J. Q. D., Marsh, E. L., Chen, S., Asirvatham, V. S., Schachtman, D. P. (2006). Characterization of the maize xylem sap proteome. J. Proteome Res. 5, 963–972. doi: 10.1021/pr050471q
Alvarez, S., Marsh, E. L., Schroeder, S. G., Schachtman, D. P. (2008). Metabolomic and proteomic changes in the xylem sap of maize under drought. Plant Cell Environ. 31, 325–340. doi: 10.1111/j.1365-3040.2007.01770.x
Amman, G. D., Schmitz, R. F. (1988). Mountain pine beetle: Lodgepole pine interactions and strategies for reducing tree losses. Ambio 17, 62–68.
Andriyas, T., Leksungnoen, N., Tor-Ngern, P. (2021). Comparison of water-use characteristics of tropical tree saplings with implications for forest restoration. Sci. Rep. 11, 1745. doi: 10.1038/s41598-021-81334-0
Assaf, G., Zieslin, N. (1996). Night water consumption by rose plants. J. Hortic. Sci. 71, 673–678. doi: 10.1080/14620316.1996.11515447
Bahrun, A., Jensen, C. R., Asch, F., Mogensen, V. O. (2002). Drought-induced changes in xylem pH, ionic composition, and ABA concentration act as early signals in field-grown maize (Zea mays l.). J. Exp. Bot. 53, 251–263. doi: 10.1093/jexbot/53.367.251
Balek, J., Pavlík, O. (1977). Sap stream velocity as an indicator of the transpirational process. J. Hydrol 34, 193–200. doi: 10.1016/0022-1694(77)90071-3
Ballester, C., Castel, J., Jiménez-Bello, M. A., Castel, J. R., Intrigliolo, D. S. (2013). Thermographic measurement of canopy temperature is a useful tool for predicting water deficit effects on fruit weight in citrus trees. Agric. Water Manage. 122, 1–6. doi: 10.1016/j.agwat.2013.02.005
Bano, A., Hansen, H., Dörffling, K., Hahn, H. (1994). Changes in the contents of free and conjugated abscisic acid, phaseic acid and cytokinins in xylem sap of drought stressed sunflower plants. Phytochemistry 37, 345–347. doi: 10.1016/0031-9422(94)85058-5
Barnett, J. R. (2004). Xylem physiology In Encyclopedia of Forest Sciences. Elsevier, 1583–1590. doi: 10.1016/B0-12-145160-7/00099-5
Bleby, T. M., Burgess, S. S. O., Adams, M. A. (2004). A validation, comparison and error analysis of two heat-pulse methods for measuring sap flow in eucalyptus marginata saplings. Funct. Plant Biol. 31, 645–658. doi: 10.1071/FP04013
Blümich, B., Blümler, P., Eidmann, G., et al. (1998). The NMR-mouse: construction, excitation, and applications. Magn Reson Imaging 16, 479–484. doi: 10.1016/S0730-725X(98)00069-1
Blümich, B., Casanova, F., Perlo, J., Presciutti, F., Anselmi, C., Doherty, B. (2010). Noninvasive testing of art and cultural heritage by mobile NMR. Acc Chem. Res. 43, 761–770. doi: 10.1021/ar900277h
Blümler, P. (2016). Proposal for a permanent magnet system with a constant gradient mechanically adjustable in direction and strength. Concepts Magn Reson Part B: Magn Reson Eng. 46, 41–48. doi: 10.1002/cmr.b.21320
Brodersen, C. R., Roddy, A. B., Wason, J. W., McElrone, A. J. (2019). Functional status of xylem through time. Annu. Rev. Plant Biol. 70, 407–433. doi: 10.1146/annurev-arplant-050718-100455
Burgess, S. S. O., Adams, M. A., Turner, N. C., Beverly, C. R., Ong, C. K., Khan, A. A. H., et al. (2001). An improved heat pulse method to measure low and reverse rates of sap flow in woody plants. Tree Physiol. 21, 589–598. doi: 10.1093/TREEPHYS/21.9.589
Bush, S. E., Hultine, K. R., Sperry, J. S., Ehleringer, J. R. (2010). Calibration of thermal dissipation sap flow probes for ring-and diffuse-porous trees. Tree Physiol. 30, 1545–1554. doi: 10.1093/treephys/tpq096
Callaghan, P. T., Coy, A., MacGowan, D., Packer, K. J., Zelaya, F. O. (1991). Diffraction-like effects in NMR diffusion studies of fluids in porous solids. Nature 351, 6326 351:467–469. doi: 10.1038/351467a0
Capitani, D., Brilli, F., Mannina, L., Proietti, N., Loreto, F. (2009). In situ investigation of leaf water status by portable unilateral nuclear magnetic resonance. Plant Physiol. 149, 1638–1647. doi: 10.1104/pp.108.128884
Casieri, C., Senni, L., Romagnoli, M., Santamaria, U., de Luca, F. (2004). Determination of moisture fraction in wood by mobile NMR device. J. Magn Reson 171, 364–372. doi: 10.1016/j.jmr.2004.09.014
Čermák, J., Deml, M., Penka, M. (1973)A new method of sap flow rate determination in trees. Available at: http://bp.ueb.cas.cz/doi/101007/BF02922390.html.
Čermák, J., Kučera, J., Nadezhdina, N. (2004). Sap flow measurements with some thermodynamic methods, flow integration within trees and scaling up from sample trees to entire forest stands. Trees 18, 529–546. doi: 10.1007/s00468-004-0339-6
Cohen, Y., Fuchs, M., Green, GC (1981). Improvement of the heat pulse method for determining sap flow in trees. Plant Cell Environ. 4, 391–397. doi: 10.1111/J.1365-3040.1981.TB02117.X
Daryanto, S., Wang, L., Jacinthe, P.-A. (2016). Global synthesis of drought effects on maize and wheat production. PloS One 11, 1–15. doi: 10.1371/journal.pone.0156362
Deng, J., Ding, G., Gao, G., et al. (2015). The sap flow dynamics and response of hedysarum scoparium to environmental factors in semiarid northwestern China. PloS One 10, e0131683-. doi: 10.1371/journal.pone.0131683
Deng, Z., Guan, H., Hutson, J., et al. (2017). A vegetation-focused soil-plant-atmospheric continuum model to study hydrodynamic soil-plant water relations. Water Resour. Res. 53, 4965–4983. doi: 10.1002/2017WR020467
de Schepper, V., van Dusschoten, D., Copini, P., Jahnke, S., Steppe, K. (2012). MRI Links stem water content to stem diameter variations in transpiring trees. J. Exp. Bot. 63, 2645–2653. doi: 10.1093/JXB/ERR445
di Tullio, V., Capitani, D., Atrei, A., Benetti, F., Perra, G., Presciutti, F., et al. (2016). Advanced NMR methodologies and micro-analytical techniques to investigate the stratigraphy and materials of 14th century sienese wooden paintings. Microchem J. 125, 208–218. doi: 10.1016/j.microc.2015.11.036
Dixon, H. H., Joly, J. (1894). On the ascent of sap. Proc. R. Soc. London 57, 3–5. doi: 10.1093/oxfordjournals.aob.a090723
Do, F. C., Isarangkool Na Ayutthaya, S., Rocheteau, A. (2011). Transient thermal dissipation method for xylem sap flow measurement: implementation with a single probe. Tree Physiol. 31, 369–380. doi: 10.1093/TREEPHYS/TPR020
Doner, L. W. (2003). “SUGAR | palms and maples,” in Encyclopedia of food sciences and nutrition (Second edition). Ed. Caballero, B. (Oxford: Academic Press), 5657–5659.
Donovan, L., Linton, M., Richards, J. (2001). Predawn plant water potential does not necessarily equilibrate with soil water potential under well-watered conditions. Oecologia 129, 328–335. doi: 10.1007/s004420100738
Doronila, A. I., Forster, M. A. (2015). Performance measurement via sap flow monitoring of three eucalyptus species for mine site and dryland salinity phytoremediation. Int. J. Phytoremediation 17, 101–108. doi: 10.1080/15226514.2013.850466
Elliott, J., Deryng, D., Müller, C., Frieler, K., Konzmann, M., Gerten, D., et al. (2014). Constraints and potentials of future irrigation water availability on agricultural production under climate change. Proc. Natl. Acad. Sci. 111, 3239–3244. doi: 10.1073/pnas.1222474110
Elsgaard, L., Børgesen, C. D., Olesen, J. E., Siebert, S., Ewert, F., Peltonen-Sainio, P., et al. (2012). Shifts in comparative advantages for maize, oat and wheat cropping under climate change in Europe. Food additives contaminants Part A Chem anal control exposure Risk Assess. 29, 1514–1526. doi: 10.1080/19440049.2012.700953
Esteves, B.d. S., Lousada L de, L., de Sousa, E. F., Campostrini, E. (2015). Advanced techniques using the plant as indicator of irrigation management. Ciec. Rural 45, 821–827. doi: 10.1590/0103-8478cr20140501
Estrada-Campuzano, G., Miralles, D. J., Slafer, G. A. (2008). Genotypic variability and response to water stress of pre- and post-anthesis phases in triticale. Eur. J. Agron. 28, 171–177. doi: 10.1016/j.eja.2007.07.005
Fayer, M. J., Gee, G. W. (2004). Neutron scattering. Encyclopedia Soils Environ. 4, 6–12. doi: 10.1016/B0-12-348530-4/00505-1
Fernández, J. E., Green, S. R., Caspari, H. W., Diaz-Espejo, A., Cuevas, M. (2008a). The use of sap flow measurements for scheduling irrigation in olive, apple and Asian pear trees and in grapevines. Plant Soil 305, 91–104. doi: 10.1007/s11104-007-9348-8
Fernández, J. E., Romero, R., Montaño, J. C., Diaz-Espejo, A., Muriel, J. L., Cuevas, M., et al. (2008b). Design and testing of an automatic irrigation controller for fruit tree orchards, based on sap flow measurements. Aust. J. Agric. Res. 59, 589–598. doi: 10.1071/AR07312
Fiscus, E. L. (2015). Water transport and balance within the plant: Resistance to water flow in roots. Limitations to Efficient Water Use in Crop Production 183–194. doi: 10.2134/1983.limitationstoefficientwateruse.c11
Forster, M. A. (2017). How reliable are heat pulse velocity methods for estimating tree transpiration? Forests 8, 350. doi: 10.3390/f8090350
Frensch, J., Hsiao, T. C. (1993). Hydraulic propagation of pressure along immature and mature xylem vessels of roots of zea mays measured by pressure-probe techniques. Planta 190, 263–270. doi: 10.1007/BF00196620
Goodger, J. Q. D., Sharp, R. E., Marsh, E. L., Schachtman, D. P. (2005). Relationships between xylem sap constituents and leaf conductance of well-watered and water-stressed maize across three xylem sap sampling techniques. J. Exp. Bot. 56, 2389–2400. doi: 10.1093/jxb/eri231
Granier, A. (1985). Une nouvelle méthode pour la mesure du flux de sève brute dans le tronc des arbres. In: Annales Des. Sci. forestières. EDP Sci. 42, 193–200. doi: 10.1051/forest:19850204
Green, S. R. (1998). Flow by the heat-pulse method. HortResearch Internal rep. 1998/22. HortResearch, Palmerston North, New Zealand, 54 p.
Gusta, L., MJ, B., Kapoor, A. C. (1975). Determination of unfrozen water in winter cereals at subfreezing temperatures. Plant Physiol. 56, 707–709. doi: 10.1104/pp.56.5.707
Halbach, K. (1980). Design of permanent multipole magnets with oriented rare earth cobalt material. Nucl. Instruments Methods 169, 1–10. doi: 10.1016/0029-554X(80)90094-4
Harris, J. M. (1961). Water-conduction in the stems of certain conifers. Nature 189, 678–679. doi: 10.1038/189678b0
Hatfield, J. L., Prueger, J. H. (2015). Temperature extremes: Effect on plant growth and development. Weather Climate Extremes 10, 4–10. doi: 10.1016/j.wace.2015.08.001
Helfter, C., Shephard, J. D., Martinez-Vilalta, J., Mencuccini, M., Hand, D. P. (2007). A noninvasive optical system for the measurement of xylem and phloem sap flow in woody plants of small stem size. Tree Physiol. 27, 169–179. doi: 10.1093/treephys/27.2.169
Hochberg, U., Windt, C. W., Ponomarenko, A., Zhang, Y.-J., Gersony, J., Rockwell, F. E., et al. (2017). Stomatal closure, basal leaf embolism, and shedding protect the hydraulic integrity of grape stems. Plant Physiol. 174, 764–775. doi: 10.1104/pp.16.01816
Homan, N. M., Windt, C. W., Vergeldt, F. J., Gerkema, E., van As, H. (2007). 0.7 and 3 T MRI and sap flow in intact trees: Xylem and phloem in action. Appl. Magn Reson 32, 157–170. doi: 10.1007/s00723-007-0014-3
Hubeau, M., Steppe, K. (2015). Plant-PET scans: In vivo mapping of xylem and phloem functioning. Trends Plant Sci. 20, 676–685. doi: 10.1016/j.tplants.2015.07.008
Huber, B. (1932). Beobachtung und messung pflanzlicher saftstrome. Ber deutsch Bot. Ges 50, 89–109. doi: 10.5917/jagh1987.41.307
Huber, C., Goertler, M., Abert, C., Bruckner, F., Groenefeld, M., Teliban, I., et al. (2018). Additive manufactured and topology optimized passive shimming elements for permanent magnetic systems. Sci. Rep. 8, 14651. doi: 10.1038/s41598-018-33059-w
Hultine, K. R., Nagler, P. L., Morino, K., Bush, S. E., Burtch, K. G., Dennison, P. E., et al. (2010). Sap flux-scaled transpiration by tamarisk (Tamarix spp.) before, during and after episodic defoliation by the saltcedar leaf beetle (Diorhabda carinulata). Agric. For. Meteorol 150, 1467–1475. doi: 10.1016/j.agrformet.2010.07.009
Hu, Q. I., Ma, X., Pan, X., Binxiang, H. (2019). Climate warming changed the planting boundaries of varieties of summer corn with different maturity levels in the north China plain. J. Appl. Meteorol Climatol 58, 2605–2615. doi: 10.1175/JAMC-D-19-0059.1
Hussain, S., Hussain, S., Qadir, T., Khaliq, A., Ashraf, U., Parveen, A., et al. (2019). Drought stress in plants: An overview on implications, tolerance mechanisms and agronomic mitigation strategies. Plant Sci. Today 6, 389–402. doi: 10.14719/pst.2019.6.4.578
Ittner, E. (1968). Der tageshang der geschwindigkeit des transpirationsstromes im stamm einer 75-jahrigen fichte. Oecol Plant 3, 177–183.
Jiang, F., Jeschke, W. D., Hartung, W. (2004). Solute flows from hordeum vulgare to the hemiparasite rhinanthus minor and the influence of infection on host and parasite nutrient relations. Funct. Plant Biol. 31, 633–643. doi: 10.1071/FP03225
Jiao, P., Wu, Z., Wang, X., Jiang, Z., Wang, Y., Liu, H., et al. (2021). Short-term transcriptomic responses of populus euphratica roots and leaves to drought stress. J. Forestry Res. 32 (2), 841–853. doi: 10.1007/s11676-020-01123-9
Jones, H. G. (2004). Irrigation scheduling: Advantages and pitfalls of plant-based methods. J. Exp. Bot. 55, 2427–2436. doi: 10.1093/jxb/erh213
Jones, T. S., Winbourne, J. B., Hutyra, L. R. (2020). Ribbonized sap flow: An emerging technology for the integration of sap flow sensor components onto a single platform. Ecosphere 11, e03135. doi: 10.1002/ecs2.3135
Kadam, N. N., Xiao, G., Melgar, R. J., Bahuguna, R. N., Quinones, C., Tamilselvan, A., et al. (2014). “Chapter three - agronomic and physiological responses to high temperature, drought, and elevated CO2 interactions in cereals,”. Ed. Sparks, D. (Academic Press) 127, 111–156. doi: 10.1016/B978-0-12-800131-8.00003-0
Kaufmann, I., Schulze-Till, T., Schneider, H. U., Zimmermann, U., Jakob, P., Wegner, L. H. (2009). Functional repair of embolized vessels in maize roots after temporal drought stress, as demonstrated by magnetic resonance imaging. New Phytol. 184, 245–256. doi: 10.1111/J.1469-8137.2009.02919.X
Kaya, M. D., Okçu, G., Atak, M., Çıkılı, Y., Kolsarıcı, Ö. (2006). Seed treatments to overcome salt and drought stress during germination in sunflower (Helianthus annuus l.). Eur. J. Agron. 24, 291–295. doi: 10.1016/j.eja.2005.08.001
Kehr, J., Buhtz, A., Giavalisco, P. (2005). Analysis of xylem sap proteins from brassica napus. BMC Plant Biol. 5, 11. doi: 10.1186/1471-2229-5-11
Kim, W., Iizumi, T., Nishimori, M. (2019). Global patterns of crop production losses associated with droughts from 1983 to 2009. J. Appl. Meteorol Climatol 58, 1233–1244. doi: 10.1175/JAMC-D-18-0174.1
Kim, H. K., Lee, S. J. (2010). Synchrotron X-ray imaging for nondestructive monitoring of sap flow dynamics through xylem vessel elements in rice leaves. New Phytol. 188, 1085–1098. doi: 10.1111/J.1469-8137.2010.03424.X
Kimura, T., Geya, Y., Terada, Y., Kose, K., Haishi, T., Gemma, H., et al. (2011). Development of a mobile magnetic resonance imaging system for outdoor tree measurements. Rev. Sci. Instruments 82. doi: 10.1063/1.3589854
King, M., Altdorff, D., Li, P., Galagedara, L., Holden, J., Unc, A. (2018). Northward shift of the agricultural climate zone under 21st-century global climate change. Sci. Rep. 8, 7904. doi: 10.1038/s41598-018-26321-8
Kirisits, T., Offenthaler, I. (2002). Xylem sap flow of Norway spruce after inoculation with the blue-stain fungus ceratocystis polonica. Plant Pathol. 51, 359–364. doi: 10.1046/j.1365-3059.2002.00722.x
Klein, Y., Abelmann, L., Gardeniers, H. (2021). Influence of the distribution of the properties of permanent magnets on the field homogeneity of magnet assemblies for mobile NMR. IEEE Transactions on Magnetics 57, 1–7. doi: 10.48550/arxiv.2103.13691
Koch, M., Missimer, T. M. (2016). Water resources assessment and management in drylands. Water 8, 239. doi: 10.3390/w8060239
Koch, K. M., Rothman, D. L., de Graaf, R. A. (2009). Optimization of static magnetic field homogeneity in the human and animal brain in vivo. Prog. Nucl. Magn. Reson. Spectrosc 54, 69–96. doi: 10.1016/j.pnmrs.2008.04.001
Köckenberger, W., Pope, J. M., Xia, Y., Jeffrey, K. R., Komor, E., Callaghan, P. T. (1997). A non-invasive measurement of phloem and xylem water flow in castor bean seedlings by nuclear magnetic resonance microimaging. Planta 201, 53–63. doi: 10.1007/BF01258680
Kozlowski, T. T., Winget, C. H. (1963). Patterns of water movement in forest trees. Bot Gazette 124, 301–311. doi: 10.1086/336210
Krishnan, H. B., Natarajan, S. S., Bennett, J. O., Sicher, R. C. (2011). Protein and metabolite composition of xylem sap from field-grown soybeans (Glycine max). Planta 233, 921–931. doi: 10.1007/s00425-011-1352-9
Ktjčera, J., Čermák, J., Penka, M. (2008). Improved thermal method of continual recording the transpiration flow rate dynamics. Biol. Plantarum 19, 6 19:413–420. doi: 10.1007/BF02922976
Lee, S. J., Kim, Y. (2008). In vivo visualization of the water-refilling process in xylem vessels using X-ray micro-imaging. Ann. Bot. 101, 595–602. doi: 10.1093/aob/mcm312
Li, D., Wang, H., Ren, Y., Wang, D. (2021). Electromagnetic design and mechanical behavior analysis of an 850 MHz all REBCO nuclear magnetic resonance magnet. IEEE Transactions on Applied Superconductivity 31. doi: 10.1109/TASC.2021.3052825
Looker, N., Martin, J., Jencso, K., Hu, J. (2016). Contribution of sapwood traits to uncertainty in conifer sap flow as estimated with the heat-ratio method. Agric. For. Meteorol 223, 60–71. doi: 10.1016/j.agrformet.2016.03.014
Malone, M. W., Yoder, J., Hunter, J. F., Espy, M. A., Dickman, L. T., Nelson, R. O., et al. (2016). In vivo observation of tree drought response with low-field NMR and neutron imaging. Front. Plant Sci. 7. doi: 10.3389/FPLS.2016.00564/BIBTEX
Maney, C., Sassen, M., Hill, S. L. L. (2022). Modelling biodiversity responses to land use in areas of cocoa cultivation. Agriculture Ecosyst. Environ. 324, 1–8. doi: 10.1016/J.AGEE.2021.107712
Marshall, D. C. (1958). Measurement of sap flow in conifers by heat transport. Plant Physiol. 33, 385–396. doi: 10.1104/pp.33.6.385
Matthews, S. N., Iverson, L. R. (2017). Managing for delicious ecosystem service under climate change: Can united states sugar maple (Acer saccharum) syrup production be maintained in a warming climate? Int. J. Biodivers Sci Ecosys Serv. Manage. 13, 40–52. doi: 10.1080/21513732.2017.1285815
McElrone, A. J., Grant, J. A., Kluepfel, D. A. (2010). The role of tyloses in crown hydraulic failure of mature walnut trees afflicted by apoplexy disorder. Tree Physiol. 30, 761–772. doi: 10.1093/treephys/tpq026
McElrone, A. J., Manuck, C. M., Brodersen, C. R., Patakas, A., Pearsall, K. R., Williams, L. E. (2021). Functional hydraulic sectoring in grapevines as evidenced by sap flow, dye infusion, leaf removal and micro-computed tomography. AoB Plants 13, plab003. doi: 10.1093/aobpla/plab003
Meixner, M., Kochs, J., Foerst, P., Windt, C. W. (2021). An integrated magnetic resonance plant imager for mobile use in greenhouse and field. J. Magn Reson 323, 106879. doi: 10.1016/J.JMR.2020.106879
Meixner, T., Manning, A. H., Stonestrom, D. A., Allen, D. M., Ajami, H., Blasch, K. W., et al. (2016). Implications of projected climate change for groundwater recharge in the western united states. J. Hydrol 534, 124–138. doi: 10.1016/j.jhydrol.2015.12.027
Merela, M., Oven, P., Serša, I., Mikac, U. (2009). A single point NMR method for an instantaneous determination of the moisture content of wood. Holzforschung 63, 348–351. doi: 10.1515/HF.2009.050
Musse, M., Leport, L., Cambert, M., Debrandt, W., Sorin, C., Bouchereau, A., et al. (2017). A mobile NMR lab for leaf phenotyping in the field. Plant Methods 13, 53. doi: 10.1186/s13007-017-0203-5
Nadezhdina, N. (1999). Sap flow index as an indicator of plant water status. Tree Physiol. 19, 885–891. doi: 10.1093/TREEPHYS/19.13.885
Nagata, A., Kose, K., Terada, Y. (2016). Development of an outdoor MRI system for measuring flow in a living tree. JMagR 265, 129–138. doi: 10.1016/J.JMR.2016.02.004
Niether, W., Jacobi, J., Blaser, W. J., Andres, C., Armengot, L. (2020). Cocoa agroforestry systems versus monocultures: A multi-dimensional meta-analysis. Environ. Res. Lett. 15, 104085. doi: 10.1088/1748-9326/abb053
Nuixe, M., Traoré, A. S., Blystone, S., Bonny, J.-M., Falcimagne, R., Pagès, G., et al. (2021). Circadian variation of root water status in three herbaceous species assessed by portable NMR. Plants (Basel) 10, 1–15. doi: 10.3390/plants10040782
Okada, T., Yamada, H., Ito, H., Yonekura, Y., Sadato, N. (2005). Magnetic field strength increase yields significantly greater contrast-to-noise ratio increase: Measured using BOLD contrast in the primary visual area. Acad. Radiol. 12, 142–147. doi: 10.1016/J.ACRA.2004.11.012
Oren, R., Sperry, J. S., Ewers, B. E., Pataki, D. E., Phillips, N., Megonigal, J. P. (2001). Sensitivity of mean canopy stomatal conductance to vapor pressure deficit in a flooded taxodium distichum l. forest: Hydraul non-hydraul effects. Oecologia 126, 1 126:21–29. doi: 10.1007/S004420000497
Ouadi, L., Bruez, E., Bastien, S., Yacoub, A., Coppin, C., Guérin-Dubrana, L., et al. (2021). Sap flow disruption in grapevine is the early signal predicting the structural, functional, and genetic responses to esca disease. Front. Plant Sci. 12. doi: 10.3389/fpls.2021.695846
Pate, J., Shedley, E., Arthur, D., Adams, M. (1998). Spatial and temporal variations in phloem sap composition of plantation-grown eucalyptus globulus. Oecologia 117, 312–322. doi: 10.1007/s004420050664
Peuke, A. D., Rokitta, M., Zimmermann, U., Schreiber, L., Haase, A. (2001). Simultaneous measurement of water flow velocity and solute transport in xylem and phloem of adult plants of ricinus communis over a daily time course by nuclear magnetic resonance spectrometry. Plant Cell \& Environ. 24, 491–503. doi: 10.1046/j.1365-3040.2001.00704.x
Peuke, Ad, Windt, C., van, A. S. H. (2006). Effects of cold-girdling on flows in the transport phloem in ricinus communis: Is mass flow inhibited? Plant Cell \& Environ. 29, 15–25. doi: 10.1111/j.1365-3040.2005.01396.x
Portmann, F. T., Döll, P., Eisner, S., Flörke, M. (2013). Impact of climate change on renewable groundwater resources: Assessing the benefits of avoided greenhouse gas emissions using selected CMIP5 climate projections. Environ. Res. Lett. 8, 1–14. doi: 10.1088/1748-9326/8/2/024023
Rabi, I. I., Millman, S., Kusch, P., Zacharias, J. R. (1939). The molecular beam resonance method for measuring nuclear magnetic moments 3 Li6, 3Li7 and 9F19. Phys. Rev. 55, 526–535. doi: 10.1103/PhysRev.55.526
Rabi, I. I., Zacharias, J. R., Millman, S., Kusch, P. (1938). A new method of measuring nuclear magnetic moment. Phys. Rev. 53, 318. doi: 10.1103/PhysRev.53.318
Raich, H., Blümler, P. (2004). Design and construction of a dipolar Halbach array with a homogeneous field from identical bar magnets: NMR Mandhalas. Concepts Magn Reson Part B Magn Reson Eng 23B, 16–25. doi: 10.1002/CMR.B.20018
Rapp, J. M., Lutz, D. A., Huish, R. D., Dufour, B., Ahmed, S., Morelli, T. L., et al. (2019). Finding the sweet spot: Shifting optimal climate for maple syrup production in north America. For. Ecol. Manage. 448, 187–197. doi: 10.1016/j.foreco.2019.05.045
Ren, R., Liu, G., Wen, M., Horton, R., Li, B., Si, B. (2017). The effects of probe misalignment on sap flux density measurements and in situ probe spacing correction methods. Agric. For. Meteorol 232, 176–185. doi: 10.1016/j.agrformet.2016.08.009
Rohula, G., Kupper, P., Räim, O., Sellin, A., Sõber, A. (2014). Patterns of night-time water use are interrelated with leaf nitrogen concentration in shoots of 16 deciduous woody species. Environ. Exp. Bot. 99, 180–188. doi: 10.1016/j.envexpbot.2013.11.017
Rokitta, M., Rommel, E., Zimmermann, U., Haase, A. (2000). Portable nuclear magnetic resonance imaging system. Rev. Sci. Instruments 71, 4257–4262. doi: 10.1063/1.1318922
Rokitta, M., Zimmermann, U., Haase, A. (1999). Fast NMR flow measurements in plants using FLASH imaging. J. Magn. Reson. 137, 29–32. doi: 10.1006/JMRE.1998.1611
Roper, J. M., Garcia, J. F., Tsutsui, H. (2021). Emerging technologies for monitoring plant health in vivo. ACS Omega 6, 5101–5107. doi: 10.1021/acsomega.0c05850
Sakuratani, T. (1981). A heat balance method for measuring water flux in the stem of intact plants. J. Agric. Meteorol 37, 9–17. doi: 10.2480/agrmet.37.9
Sakuratani, T., Abe, J. (1985). A heat balance method for measuring water flow rate in stems of intact plants and its application to sugarcane plants. JARQ. Japan Agricultural Research Quarterly. 19, 92–97.
Samarah, N. H. (2005). Effects of drought stress on growth and yield of barley. Agron. Sustain Dev. 25, 145–149. doi: 10.1051/agro:2004064
Sardans, J., Peñuelas, J., Lope-Piedrafita, S. (2010). Changes in water content and distribution in quercus ilex leaves during progressive drought assessed by in vivo 1H magnetic resonance imaging. BMC Plant Biol. 10, 1–12. doi: 10.1186/1471-2229-10-188
Scheenen, T. W. J., van Dusschoten, D., de Jager, P. A., van As, H. (2000). Quantification of water transport in plants with NMR imaging. J. Exp. Bot. 51, 1751–1759. doi: 10.1093/jexbot/51.351.1751
Scheenen, T. W. J., Vergeldt, F. J., Heemskerk, A. M., van As, H. (2007). Intact plant magnetic resonance imaging to study dynamics in long-distance sap flow and flow-conducting surface area. Plant Physiol. 144, 1157. doi: 10.1104/PP.106.089250
Shaw, T. M., Elsken, R. H. (1956). Moisture determination, determination of water by nuclear magnetic absorption in potato and apple tissue. J. Agric. Food Chem. 4, 162–164. doi: 10.1021/jf60060a008
Snyder, K. A., Richards, J. H., Donovan, L. A. (2003). Night-time conductance in C3 and C4 species: Do plants lose water at night? J. Exp. Bot. 54, 861–865. doi: 10.1093/JXB/ERG082
Soltner, H., Blümler, P. (2010). Dipolar halbach magnet stacks made from identically shaped permanent magnets for magnetic resonance. Concepts Magn Reson Part A 36A, 211–222. doi: 10.1002/CMR.A.20165
Steppe, K., de Pauw, D. J. W., Doody, T. M., Teskey, R. O. (2010). A comparison of sap flux density using thermal dissipation, heat pulse velocity and heat field deformation methods. Agric. For. Meteorol 150, 1046–1056. doi: 10.1016/J.AGRFORMET.2010.04.004
Steppe, K., Vandegehuchte, M. W., Tognetti, R., Mencuccini, M. (2015). Sap flow as a key trait in the understanding of plant hydraulic functioning. Tree Physiol. 35, 341–345. doi: 10.1093/treephys/tpv033
Suárez, J. C., Casanoves, F., Bieng, M. A. N., Melgarejo, L. M., di Rienzo, J. A., Armas, C. (2021). Prediction model for sap flow in cacao trees under different radiation intensities in the western Colombian Amazon. Sci. Rep. 11, 10512. doi: 10.1038/s41598-021-89876-z
Sun, Q., Rost, T. L., Matthews, M. A. (2006). Pruning-induced tylose development in stems of current-year shoots of vitis vinifera (Vitaceae). Am. J. Bot. 93, 1567–1576. doi: 10.3732/ajb.93.11.1567
Sun, Q., Sun, Y., Walker, M. A., Labavitch, J. M. (2013). Vascular occlusions in grapevines with pierce’s disease make disease symptom development worse. Plant Physiol. 161, 1529–1541. doi: 10.1104/pp.112.208157
Tatarinov, F. A., Kučera, J., Cienciala, E. (2005). The analysis of physical background of tree sap flow measurement based on thermal methods. Meas Sci. Technol. 16, 1157–1169. doi: 10.1088/0957-0233/16/5/016
Terada, Y., Horikawa, Y., Nagata, A., Kose, K., Fukuda, K. (2020). Dynamics of xylem and phloem sap flow in an outdoor zelkova tree visualized by magnetic resonance imaging. Tree Physiol. 40, 290–304. doi: 10.1093/treephys/tpz120
Tyree, M. T., Zimmermann, M. H. (2002). Xylem structure and the ascent of sap, 2nd edn (Berlin, Heidelberg: Springer). doi: 10.1007/978-3-662-04931-0
Umebayashi, T., Fukuda, K., Haishi, T., Sotooka, R., Zuhair, S., Otsuki, K. (2011). The developmental process of xylem embolisms in pine wilt disease monitored by multipoint imaging using compact magnetic resonance imaging. Plant Physiol. 156, 943–951. doi: 10.1104/pp.110.170282
van As, H., Reinders, J. E. A., de Jager, P. A., van de Sanden, P. A. C. M., Schaafsma, T. J. (1994). In situ plant water balance studies using a portable NMR spectrometer. J. Exp. Bot. 45, 61–67. doi: 10.1093/jxb/45.1.61
van As, H., Schaafsma, T. J. (1984). Noninvasive measurement of plant water flow by nuclear magnetic resonance. Biophys. J. 45, 469–472. doi: 10.1016/S0006-3495(84)84170-3
van As, H., Scheenen, T., Vergeldt, F. J. (2009). MRI Of intact plants. Photosynth Res. 102, 213. doi: 10.1007/S11120-009-9486-3
Vandegehuchte, M. W., Steppe, K. (2012). Sapflow+: A four-needle heat-pulse sap flow sensor enabling nonempirical sap flux density and water content measurements. New Phytol. 196, 306–317. doi: 10.1111/j.1469-8137.2012.04237.x
Vandegehuchte, M. W., Steppe, K. (2013). Sap-flux density measurement methods: working principles and applicability. Funct. Plant Biol. 40, 213–223. doi: 10.1071/FP12233
Vicente, M. A., Fernando Gallardo, J., Moreno, G., González, M. I. (2003). Comparison of soil water-contents as measured with a neutron probe and time domain reflectometry in a Mediterranean forest (“Sierra de gata”, central Western Spain). Ann. For Sci. 60, 185–193. doi: 10.1051/forest:2003011
Vidana Gamage, D. N., Vasava, H. B., Strachan, I. B., Adamchuk, V. I., Biswas, A.. (2021). Comparison of heating strategies on soil water measurement using actively heated fiber optics on contrasting textured soils. Sensors (Basel) 21, 1–18. doi: 10.3390/s21030962
Vieweg, G. H., Ziegler, H. (1960). Thermoelektrische registrierung der geschwindigkeit des transpirationsstromes. Ber Dtsch Bot. Ges 73, 221–226.
Wada, Y., Wisser, D., Eisner, S., Flörke, M., Gerten, D., Haddeland, I., et al. (2013). Multimodel projections and uncertainties of irrigation water demand under climate change. Geophys Res. Lett. 40, 4626–4632. doi: 10.1002/grl.50686
Windt, C. W., Blümler, P. (2015). A portable NMR sensor to measure dynamic changes in the amount of water in living stems or fruit and its potential to measure sap flow. Tree Physiol. 35, 366–375. doi: 10.1093/treephys/tpu105
Windt, C. W., Gerkema, E., van As, H. (2009). Most water in the tomato truss is imported through the xylem, not the phloem: A nuclear magnetic resonance flow imaging study. Plant Physiol. 151, 830–842. doi: 10.1104/PP.109.141044
Windt, C. W., Nabel, M., Kochs, J., Jahnke, S., Schurr, U.. (2021). A mobile NMR sensor and relaxometric method to non-destructively monitor water and dry matter content in plants. Front. Plant Sci. 12. doi: 10.3389/FPLS.2021.617768/BIBTEX
Windt, C. W., Soltner, H., van Dusschoten, D., Blümler, P. (2011). A portable halbach magnet that can be opened and closed without force: The NMR-CUFF. J. Magn Reson 208, 27–33. doi: 10.1016/j.jmr.2010.09.020
Windt, C. W., Vergeldt, F. J., de Jager, P. A., van As, H. (2006). MRI Of long-distance water transport: A comparison of the phloem and xylem flow characteristics and dynamics in poplar, castor bean, tomato and tobacco. Plant Cell Environ. 29, 1715–1729. doi: 10.1111/J.1365-3040.2006.01544.X
Wullschleger, S. D., Childs, K. W., King, A. W., Hanson, P. J. (2011). A model of heat transfer in sapwood and implications for sap flux density measurements using thermal dissipation probes. Tree Physiol. 31, 669–679. doi: 10.1093/treephys/tpr051
Yamaoka, Y., Swanson, R. H., Hiratsuka, Y. (1990). Inoculation of lodgepole pine with four blue-stain fungi associated with mountain pine beetle, monitored by a heat pulse velocity (HPV) instrument. Can. J. For. Res. 20, 31–36. doi: 10.1139/x90-005
Yang, X.g., Liu, Z.j., Chen, F. (2011). The possible effect of climate warming on northern limits of cropping system and crop yield in China. Agric. Sci. China 10, 585–594. doi: 10.1016/S1671-2927(11)60040-0
Zhang, J., Zhang, S., Cheng, M., Jiang, H., Zhang, X., Peng, C., et al. (2018). Effect of drought on agronomic traits of rice and wheat: A meta-analysis. Int. J. Environ. Res. Public Health 15, 839. doi: 10.3390/ijerph15050839
Keywords: magnetic apparatus, nuclear magnetic resonance, plant, sap flow, stem, xylem
Citation: Kumar R, Hosseinzadehtaher M, Hein N, Shadmand M, Jagadish SVK and Ghanbarian B (2022) Challenges and advances in measuring sap flow in agriculture and agroforestry: A review with focus on nuclear magnetic resonance. Front. Plant Sci. 13:1036078. doi: 10.3389/fpls.2022.1036078
Received: 03 September 2022; Accepted: 28 September 2022;
Published: 08 November 2022.
Edited by:
Mansour Ghorbanpour, Arak University, IranReviewed by:
Javad Hadian, University of the Fraser Valley, CanadaNyong Princely Awazi, The University of Bamenda, Cameroon
Copyright © 2022 Kumar, Hosseinzadehtaher, Hein, Shadmand, Jagadish and Ghanbarian. This is an open-access article distributed under the terms of the Creative Commons Attribution License (CC BY). The use, distribution or reproduction in other forums is permitted, provided the original author(s) and the copyright owner(s) are credited and that the original publication in this journal is cited, in accordance with accepted academic practice. No use, distribution or reproduction is permitted which does not comply with these terms.
*Correspondence: Behzad Ghanbarian, ghanbarian@ksu.edu