- 1Departamento de Biología Vegetal y Ecología, Universidad de Sevilla, Seville, Spain
- 2Laboratorio Nacional de Análisis y Síntesis Ecológica, Escuela Superior de Desarrollo Sustentable, Universidad Autónoma de Guerrero – CONACYT, Chilpancingo de los Bravo, Mexico
The Mediterranean region is one of the most important worldwide hotspots in terms of number of species and endemism, and multiple hypotheses have been proposed to explain how diversification occurred in this area. The contribution of different traits to the diversification process has been evaluated in different groups of plants. In the case of Centaurium (Gentianaceae), a genus with a center of diversity placed in the Mediterranean region, polyploidy seems to have been an important driver of diversification as more than half of species are polyploids. Moreover, ploidy levels are strongly geographically structured across the range of the genus, with tetraploids distributed towards more temperate areas in the north and hexaploids in more arid areas towards the south. We hypothesize that the diversification processes and biodiversity patterns in Centaurium are explained by the coupled formation of polyploid lineages and the colonization of different areas. A MCC tree from BEAST2 based on three nuclear DNA regions of a total of 26 taxa (full sampling, of 18 species and 8 subspecies) was used to perform ancestral area reconstruction analysis in “BioGeoBEARS.” Chromosome evolution was analyzed in chromEvol and diversification in BAMM to estimate diversification rates. Our results suggest that two major clades diverged early from the common ancestor, one most likely in the western Mediterranean and the other in a widespread area including west and central Asia (but with high uncertainty in the exact composition of this widespread area). Most ancestral lineages in the western clade remained in or around the western Mediterranean, and dispersal to other areas (mainly northward and eastward), occurred at the tips. Contrarily, most ancestral lineages in the widespread clade had larger ancestral areas. Polyploidization events in the western clade occurred at the tips of the phylogeny (with one exception of a polyploidization event in a very shallow node) and were mainly tetraploid, while polyploidization events occurred in the widespread clade were at the tips and in an ancestral node of the phylogeny, and were mainly hexaploid. We show how ancestral diploid lineages remained in the area of origin, whereas recent and ancestral polyploidization could have facilitated colonization and establishment in other areas.
Introduction
Some areas on Earth have experienced high radiations and become especially rich in the number of species compared to others. These areas of high species richness and elevated percentage of endemism are known as biodiversity hotspots (Myers, 1988). Many factors have been claimed as drivers of increased biodiversity in hotspots, such as long-standing climatic and environmental stability (Fordham et al., 2019), strong spatial variability leading to habitat heterogeneity (e.g., Noroozi et al., 2018), specialized biotic interactions (e.g., Duffy and Johnson, 2017), and prevalence of reproductive systems promoting adaptive novel genetic combinations (Barrett, 2002). One of the drivers which have been proposed is polyploidization, which facilitates both reproductive isolation and adaptation to novel environments (Tate et al., 2005).
Polyploidy has been described to be an important process leading to speciation in plants (Stebbins, 1947; Tate et al., 2005). This mechanism consists of acquiring new sets of chromosomes by hybridization and duplication of the genome (allopolyploidy) or by self-duplication of the whole genome (autopolyploidy). This is a common process; in angiosperms, for example, most species have experienced one or more rounds of polyploidization and subsequent post-polyploid diploidization (Wendel, 2015; Escudero and Wendel, 2020), and at least 47% of species have undergone a recent polyploidy event (Wood et al., 2009). This process can drive speciation promoting reproductive isolation or increasing the probability for new genetic combinations as the species’ genetic content increases. Additionally, polyploids have been demonstrated to tolerate wider climatic and ecological conditions and colonize new areas, facilitated by their increased phenotypic plasticity and higher genetic diversity (see Thompson, 2020).
With about 25,000 plant species, of which about 50% are endemic, the Mediterranean basin region has been considered among the most important biodiversity hotspots (Myers et al., 2000). The Mediterranean climate, rugged topography, heterogeneous geological and geomorphological setting, the isolation provided by many mountains and islands, and historical variations have been considered important causal factors for the huge number of species and fast diversification in the region (Buerki et al., 2012; Rundel et al., 2016). For example, with respect to geography, the formation of the current insular system, especially after the Messinian salinity crisis (5.96–5.33 Ma; Duggen et al., 2003) played a key role on the formation of new species by isolation (Krijgsman et al., 1999; Buerki et al., 2012). Moreover, Anatolia, the Balkans, and the Iberian and Italian peninsulas, have served as refugia for many species during the Quaternary glaciations (Hewitt, 2004; Míguez et al., 2017; Thompson, 2020). Thus, the region became not only an important refuge for many animals and plants but also a source area for the colonization of other regions during the Holocene, after glaciations (Hewitt, 2004; Ali et al., 2019; Thompson, 2020). Climatic oscillations during the Quaternary also led species’ ranges to contract and expand, producing diversification (Avise, 2000; Maguilla et al., 2017; Schneeweiss et al., 2017). Many studies have attempted to understand how diversification takes place in the Mediterranean region using different study cases (e.g., Barres et al., 2013; Maguilla et al., 2017; Affenzeller et al., 2018, etc.). The importance of different traits in the process of diversification depends on the organism under study, and many traits have been proposed to have an impact on speciation or extinction rates (Fitzjohn, 2010).
The genus Centaurium Hill (Gentianaceae Juss.), with ca. 26 taxa (18 species and eight subspecies, Mansion and Struwe, 2004; Díaz-Lifante, 2012) has its center of diversity in the Mediterranean basin, although the range of the genus includes Asia, Europe, North-central Africa, and North America (Mansion and Struwe, 2004). In addition to their natural distribution, members of the genus have been introduced into areas of South America, Australia, and New Zealand with similar climates (Mansion and Struwe, 2004). In this genus, 60% of taxa are polyploids (Zeltner, 1970; Mansion et al., 2005), which have been demonstrated to be important in the evolution of Centaurium (Mansion et al., 2005). More interestingly, ploidy levels in the genus seem to conform to a geographical pattern, with diploid species (2n) close to the Mediterranean basin, tetraploids (4n) mainly in northern Europe and eastern Asia, and hexaploids (6n) generally distributed in India, the Arabic Peninsula, and southwestern Mediterranean basin reaching the Canary Islands (Mansion et al., 2005). The hexaploids (50% of polyploid taxa) have been proposed to be allopolyploids, while the tetraploids have been suggested to be autopolyploids (Zeltner, 1970; Mansion et al., 2005). However, in many cases, it is very difficult to distinguish allopolyploids from autopolyploids because it is difficult to determine whether gene flow during the course of evolution has occurred between two species or two lineages within the same species. So far, only one clear case of allopolyploidy has been reported for the genus Centaurium, in Centaurium discolor, in which the two parental species from two different main clades, Centaurium maritium and Centaurium tenuiflorum formed a new allopolyploid species (Guggisberg et al., 2006).
In this study, we aim to reconstruct the biogeographic history of Centaurium as well as its diversification and chromosome evolution in order to understand the geographic pattern of polyploidy and how polyploidization contributed to the evolution, dispersal, and diversification of the genus from its origin in concert with the range expansion of the genus. We hypothesize that the diversification processes and biodiversity patterns in Centaurium are explained by the coupled formation of polyploid lineages and the colonization of new areas. To our knowledge, this is a very innovative study with only one recently published study addressing the same macroevolutionary hypothesis but with a different approach (Han et al., 2020).
Materials and Methods
Sampling
Samples were collected in the field and from the following herbaria: MA, MGC, NEU, SANT, SEV, and VAL (Thiers, 2015) by Jiménez-Lobato et al. (2019). A Maximum Clade Credibility (MCC) tree constructed by Jiménez-Lobato et al. (2019) using BEAST 2.4.0 (Bouckaert et al., 2014) was used as input for our analyses after removing outgroup species. This MCC tree was based on nuclear DNA regions CPR1, ETS, and ITS (see details in Jiménez-Lobato et al., 2019) and includes all known taxa within genus Centaurium following taxonomic treatment by Mansion and Struwe (2004), and Díaz-Lifante (2012) for species occurring in the Iberian Peninsula. In total, 26 taxa (18 species and 8 subspecies) were considered for the genus.
Ancestral Area Reconstruction
The package BioGeoBEARS 1.1 (Matzke, 2013) implemented in R 3.2.2 (R Core Team, 2015) was used for the ancestral area reconstruction analyses after coding species’ occurrences within six biogeographic areas (see species scoring in Figure 1): (1) Western Mediterranean, (2) Eastern Mediterranean, (3) northern Europe, (4) northern Africa excluding the Mediterranean region, (5) western and central Asia, and (6) North America. These areas were delimited based on climatic and paleogeographic data following Buerki et al. (2012) with modifications according to species’ distribution patterns in Centaurium. The main modification was the split of the Mediterranean into two areas; the western and the eastern Mediterranean, as proposed by other authors (e.g., Takhtajan, 1986) and because many species in the genus are distributed only in the western Mediterranean. Introduced areas were excluded from the scoring of the species’ occurrence matrix.
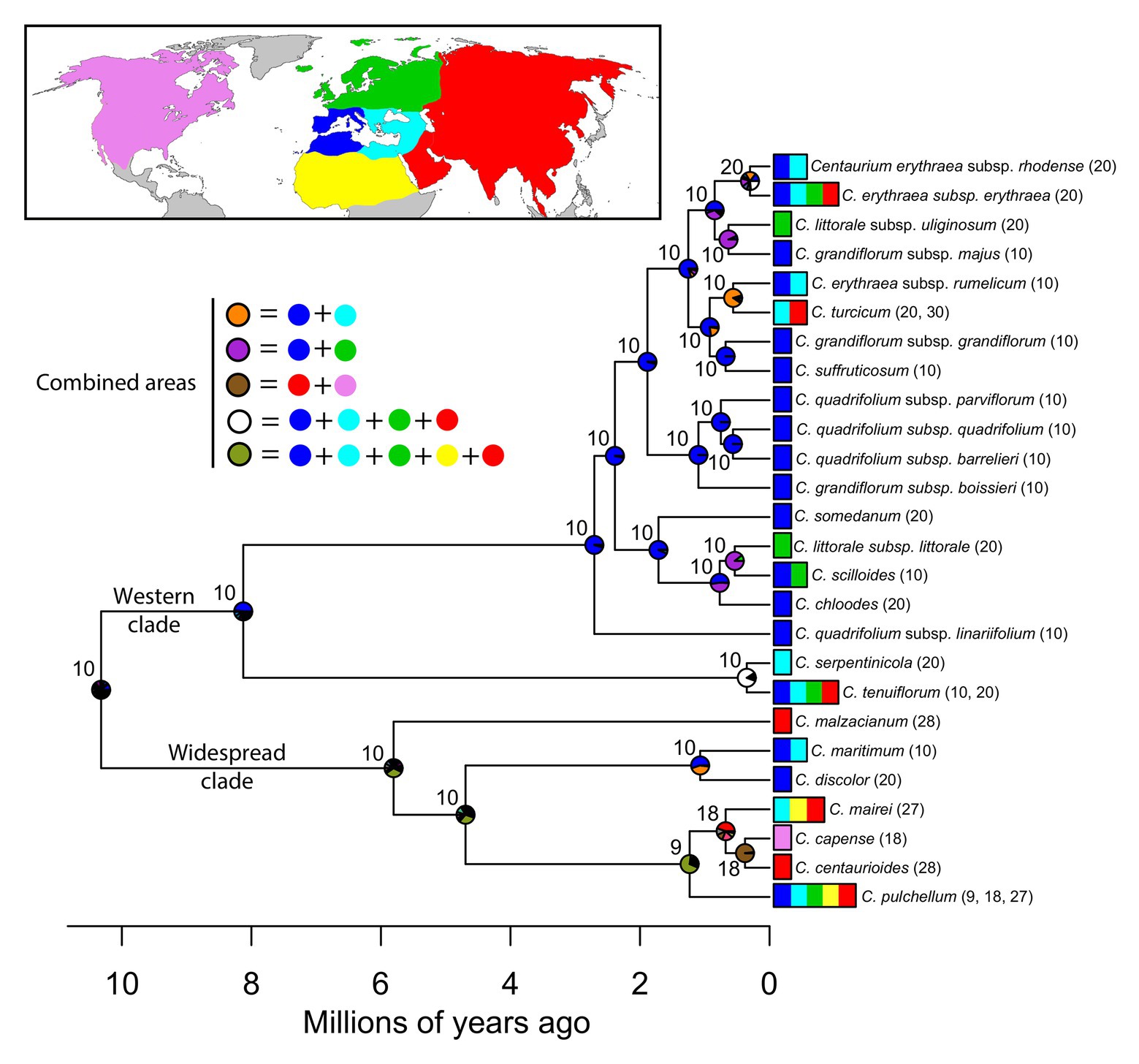
Figure 1. Ancestral area reconstruction under constrained DEC model in Centaurium resulting from BioGeoBEARS analysis (Matzke, 2013). Node charts represent the probability of the occurrence of the most recent common ancestor (MRCA) of that node in the area represented by the same color on the map, and combined areas are represented by colors in the legend. Node numbers indicate the reconstructed haploid chromosome numbers retrieved by ChromEvol (Glick and Mayrose, 2014). Tip labels indicate species names and the area where each species occurs following colors in the map. Haploid chromosome number (n, in parenthesis) and ploidy levels for the species are also indicated. Lower time-scale shows Millions of years ago, from the origin of the genus to the present.
Three biogeographic models were tested in BioGeoBEARS: Dispersal-extinction-cladogenesis (DEC; Richard and Stephen, 2008), dispersal-vicariance-like (DIVA; Ronquist, 1997), and BayArea-like (Bayesian analysis; Landis et al., 2013). Range expansion and contraction (parameters “d” and “e”) were allowed to vary during the analyses. Additionally, biogeographic reconstructions were done including founder events or jump dispersal as an additional free parameter (“j”). This reached a total of six combinations of models tested. Moreover, all these analyses were repeated including a connectivity matrix based on Hilpold et al. (2014), where dispersal probability between areas was set to one for adjacent areas, 0.5 for areas with an intermediate area between them, and 0.1 for areas separated by more than one area (Supplementary Table 1). In these cases, the parameter “w” was considered as a free parameter to reduce subjectivity induced by the assignment of a value for dispersal between geographic areas. The Akaike Information Criterion weight (AICw; Akaike, 1974) was used to compare among the 12 model combinations, selecting the best fit model. As broadly discussed by Ree and Sanmartín (2018), models that include jump dispersal (“j”) tend to overestimate cladogenetic dispersal (or jump dispersal) as the main mechanism explaining species’ distribution, considering in many cases null anagenetic rates for the model. For this reason, models including the parameter “j” were interpreted with caution and were included mainly to test whether this parameter significantly affected the results. Additionally, to avoid phylogenetic uncertainty bias, we ran in BioGeoBEARS the best fitting model in 100 independent post-burn-in trees from BEAST, and later estimated average probabilities from all trees.
Finally, biogeographic stochastic mapping (BSM; Matzke, 2014) was done in BioGeoBEARS after 50 runs to characterize the number and nature of biogeographical events in the best fitting model. We tested the confidence of the BSM by comparing states probabilities with those obtained under a maximum likelihood (ML) model.
Chromosome Evolution
The evolution of the chromosome number across the phylogeny was predicted using ChromEvol 2.0 (Mayrose et al., 2010; Glick and Mayrose, 2014). Haploid chromosome numbers (n) for all species were obtained from Mansion et al. (2005) and Díaz-Lifante (2012). In the case of species with different ploidy levels, the lowest chromosome number was assumed to be ancestral for the analyses (although only three out of 26 taxa had more than one ploidy level: Centaurium pulchellum, Centaurium turcicum, and C. tenuiflorum). A total of 10 models of chromosome evolution were tested. The analyses were performed following Escudero et al. (2014; but see also Glick and Mayrose, 2014; Aparicio et al., 2019). Akaike’s information criterion (AIC; Akaike, 1974) was used for comparing and choosing the best fitting model of chromosome evolution, which was later used for the reconstruction and plotting of chromosome numbers in the phylogeny (Glick and Mayrose, 2014).
Diversification Analyses
Diversification rates across the phylogeny were estimated using Bayesian analyses of macroevolutionary mixtures (BAMM; Rabosky et al., 2013, 2014a; Shi and Rabosky, 2015). These analyses were done using BAMM 2.5 in the R package BAMMtools (Rabosky et al., 2014b), allowing changes over time and shifts in diversification rates for five million generations. Markov-Chain Monte Carlo (MCMC) convergence was checked using the R package coda (Plummer et al., 2006), and results of the model with the highest posterior probability (PP) were summarized using BAMMtools.
Pagel’s Model for Correlated Evolution
Dependent or independent evolution modes of polyploidy and geographic distribution were also tested using Pagel’s method (Pagel, 1994) using the function “fitPagel” from the R package phytools (Revell, 2012). This function searches for correlated evolution of two binary traits. Species and subspecies were coded as diploids against polyploids (including with more than one level of ploidy). Regarding geographic distribution, first, species and subspecies were coded as narrow distributed if they only occur in one area or widespread if they occur in two or more areas. Second, the narrow distributed were coded as exclusively within the area of origin vs. dispersed outside the area of origin, following the biogeographic reconstruction obtained in BioGeoBEARS. For the western clade, the interpretation of the western Mediterranean basin as ancestral range was of high confidence. However, the uncertainty of the ancestral area in the widespread clade was very high. The species with narrow distributions Centaurium malzacianum, C. discolor, and Centaurium centauroides were interpreted as been in their original range as inferred ancestral ranges were widespread ranges including the range of these narrow distributed species. The species Centaurium capense from North America was coded as out of the ancestral range based on the reconstruction (as North America was excluded as the possible ancestral distribution in the deeper nodes of the widespread clade). We grouped the widespread species and narrow species out of the ancestral range in one category and the narrow species in the ancestral range in another category. Using these scorings, we tested for the correlation between polyploidy and widespread distribution and/or the expansion to new areas outside of the area of origin of the species or subspecies. We ran models with different rates of transitions between character states (“ARD”), assuming null rates of transition from a polyploid state to a diploid state. Additionally, we performed all analyses using three different models: (i) assuming that the variable polyploidy depends on distribution, (ii) that distribution depends on polyploidy, or (iii) that the two variables depend on each other.
Results
Ancestral Areas
The best biogeographic model based on AICc weights (Akaike, 1974) was DEC + Jc (AICc weight = 0.98; Table 1; Supplementary Figure 1); this was a constrained DEC model using the dispersal matrix and setting “j” as a free parameter. Nevertheless, given the overestimation of founder events explained above, we interpreted this model with caution. The constrained DEC model (DECc; including dispersal matrix), was the best fitting model with an AICc weight of 0.82 when comparing models without “j” as a free parameter (Table 1). In this case, the model showed an extinction rate (“e”) of 0.019 events/Myr, and an anagenetic dispersal rate (“d”) of 0.085 events/Myr (Table 1). These results were almost identical to the model obtained under the DECc model in BioGeoBEARS in 100 independent post-burn-in trees (see summarize result in Supplementary Figure 2; accordingly, we used the MCC tree results from here).
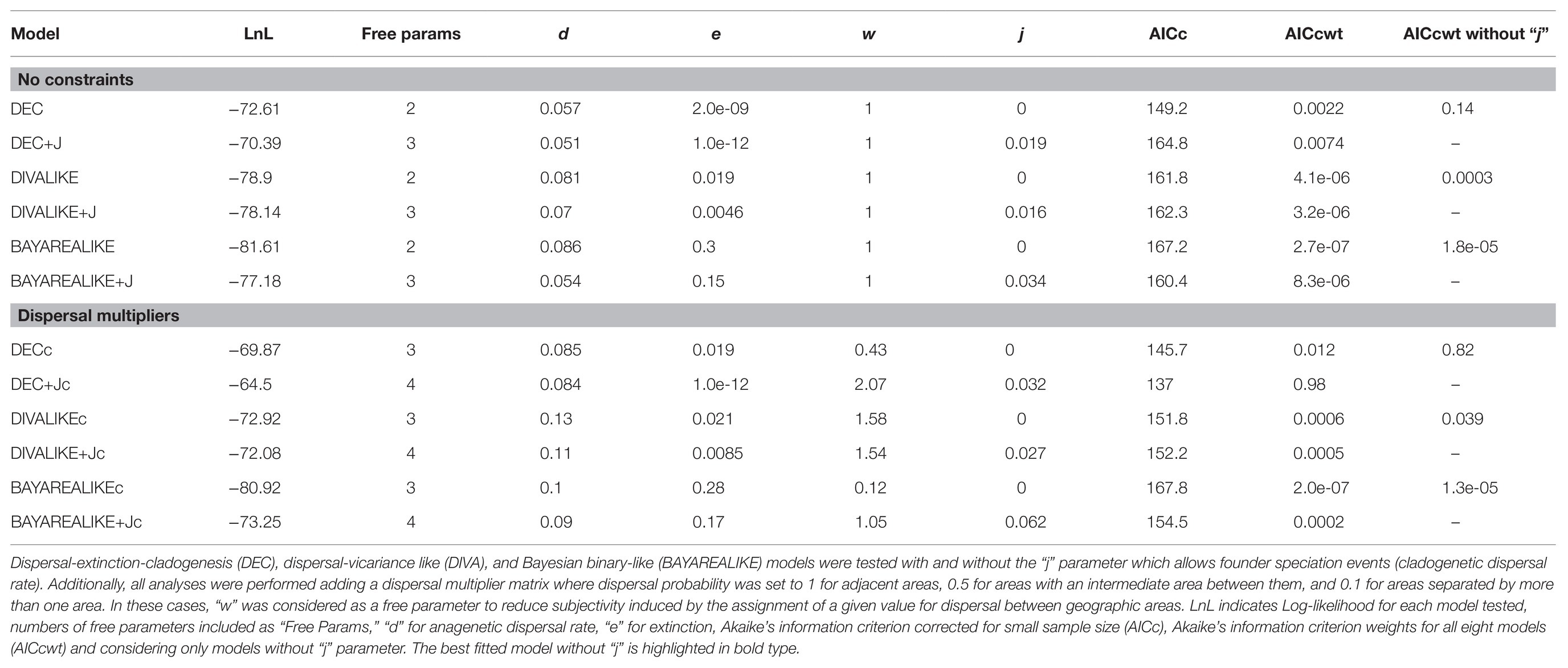
Table 1. Biogeographical models tested in BioGeoBEARS (Matzke, 2013) for Centaurium.
After the most recent common ancestor (MRCA) of the whole genus Centaurium around 10 million years ago (Ma), this phylogeny showed an early split into two major clades (Figure 1). For the first major clade (hereafter the western clade) comprising most species and subspecies from the western Mediterranean and some species and subspecies from surrounding areas (the rest of the Palaearctic), the MRCA was most likely predicted to be from the western Mediterranean (Figure 1; other possible ancestral ranges were unlikely and included western Mediterranean as part of the ancestral distribution). The origin of the MRCA for the second major clade (hereafter the widespread clade) was unresolved, as occurred with other internal nodes within this clade, suggesting wider ancestral areas. This second major clade was composed of C. capense, C. centaurioides, C. discolor, Centaurium mairei, C. malzacianum, Centaurium maritimum and the broadest distributed species in the genus, C. pulchellum, which is present in all codified areas (but North America). In the evolution of Centaurium, anagenetic dispersal was apparently the most important mechanism explaining the current distribution and biogeographic history of the genus (a mean of 21.28 ± 2.02 events after 50 BSMs; Figure 2). The area of origin of most Centaurium species and subspecies, the western Mediterranean, acted as a source for many dispersals to other areas (41.64% of all dispersal events; Figures 1, 3). Most of these dispersals took place from the western Mediterranean to the eastern Mediterranean and northern Europe (a mean of 3.64 and 3.46 events, respectively; Figure 3), and most of them took place towards the tips of the phylogeny (Figure 1). This mechanism was followed in importance by within-area speciation, which showed a mean of 12.62 ± 1.48 events after 50 BSM runs. Less important were subset within-area speciation (a mean of 8.18 ± 1.72 events) and vicariance (4.2 ± 1.55 events; Figure 2). Finally, a comparison of state probabilities obtained using Bayesian stochastic mapping and ML approaches were compared to test the suitability of the model, obtaining an R2 of 0.985 (Supplementary Figure 3), which confirmed that states predicted after the 50 BSMs were strongly congruent with a ML approach.
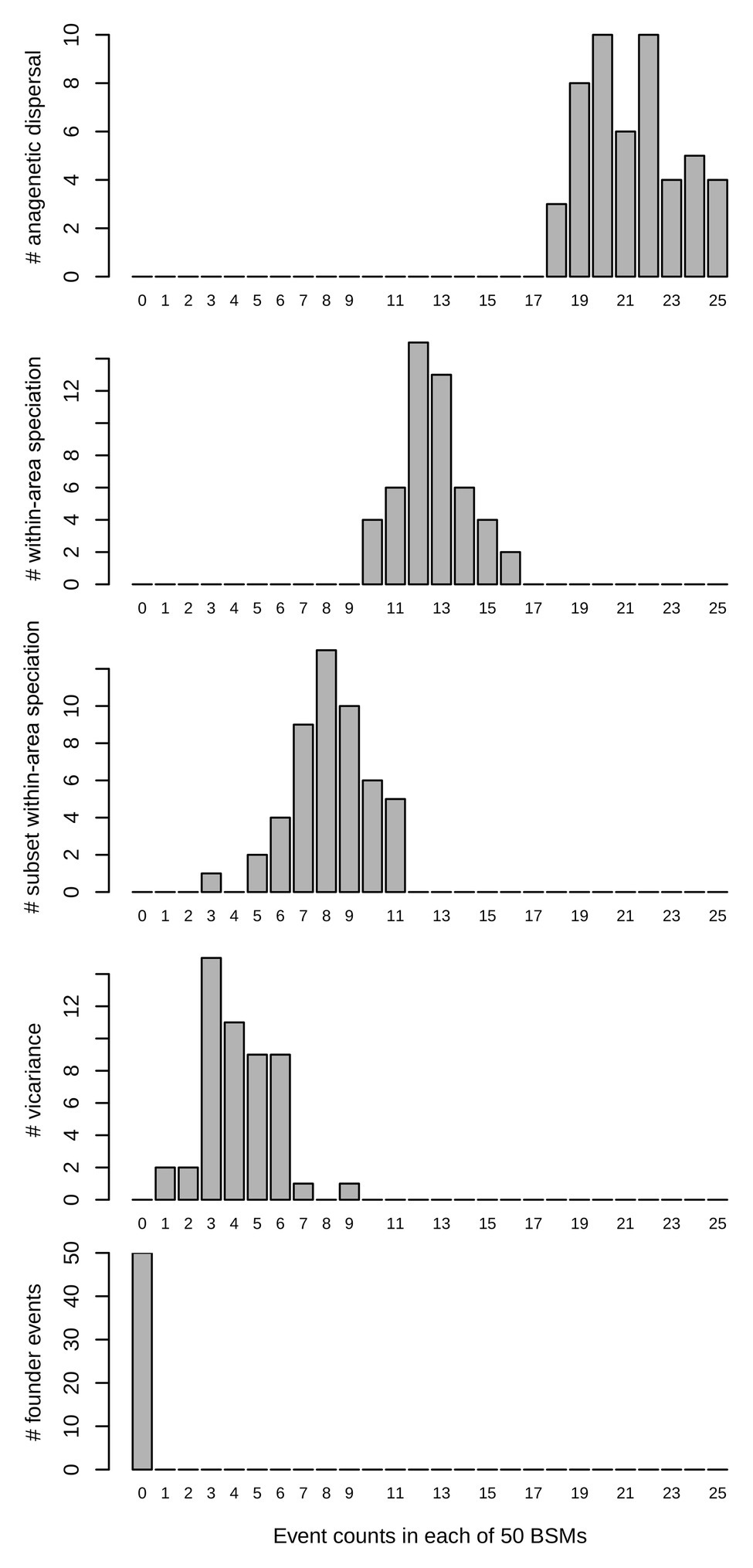
Figure 2. Biogeographical events count after 50 biogeographic stochastic mappings (BSMs) in the best fitting model obtained in BioGeoBEARS (constrained DEC model) in Centaurium. The horizontal (x) axis represents the number of events, and the vertical (y) axis the number of BSM analyses.
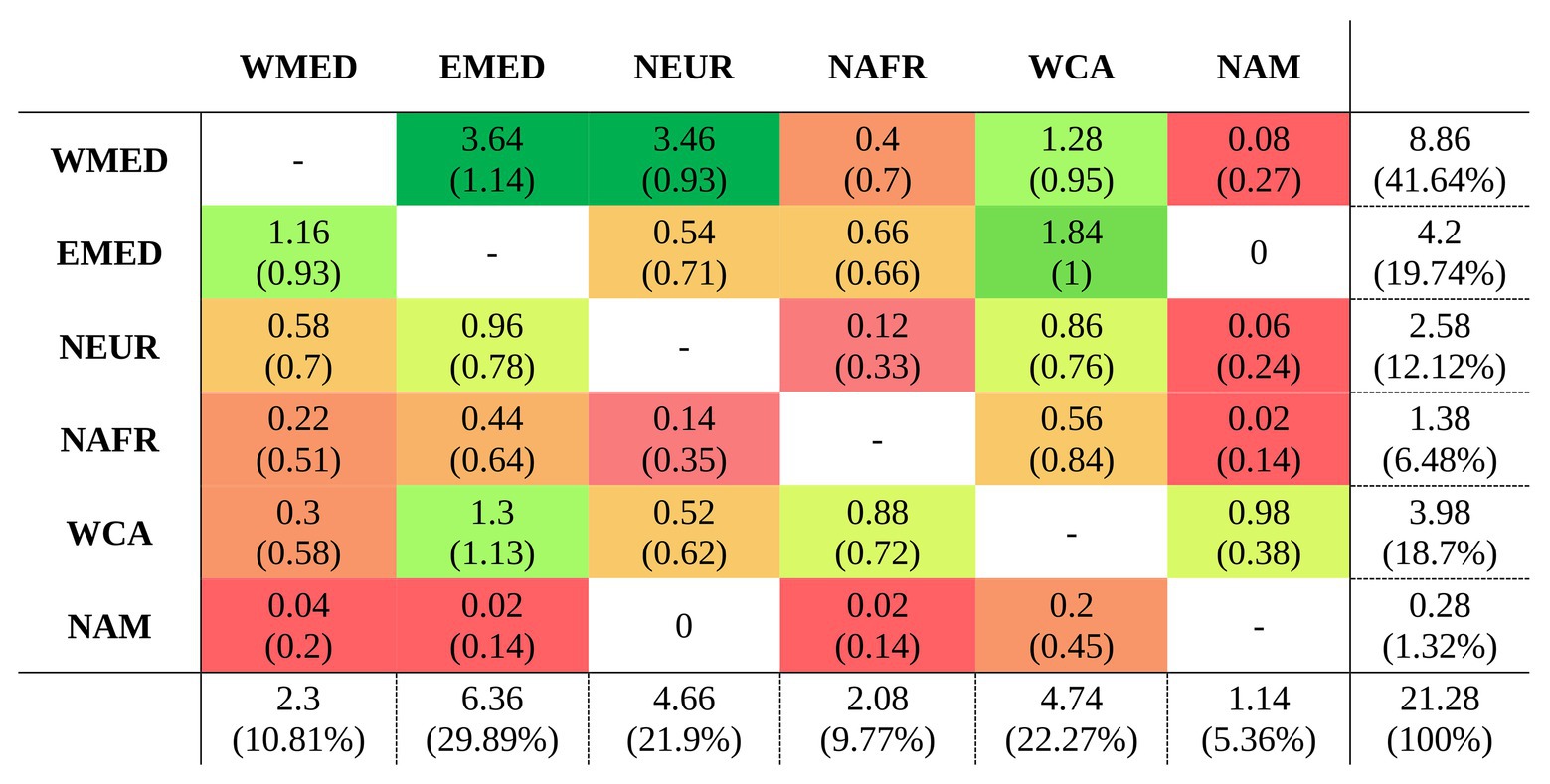
Figure 3. Average number of dispersals and standard deviation (in parenthesis) after 50 BSMs obtained from BioGeoBEARS (Matzke, 2013) under the constrained DEC model in Centaurium. Source and sink areas are indicated in the y and x axes respectively, codified as follows: WMED for western Mediterranean; EMED, eastern Mediterranean; NEUR, northern Europe; NAFR, northern Africa; WCA, west and central Asia; and NAM for North America. The frequency of each event is also indicated by the cell color (green colors for more frequent events, and warmer colors for infrequent or null events). The last row and column summarize the dispersal events or destination of each area, respectively, indicating also the percentage of all events.
Diversification Rates
The BAMM analyses showed that diversification rates were slightly increasing towards the present in Centaurium (Figure 4). A scenario without shifts in diversification rates was the most plausible (probability = 0.811), followed by a scenario with one shift in diversification rates, though this scenario had a probability of only 0.137. The speciation rate for the most supported model (0-shifts) was 0.654 lineage per million years (lin./Myr; lam1), with an extinction rate of 0.546 lin./Myr (mu1) and speciation growth parameter of 0.018 lin./Myr (lam2; Figure 4).
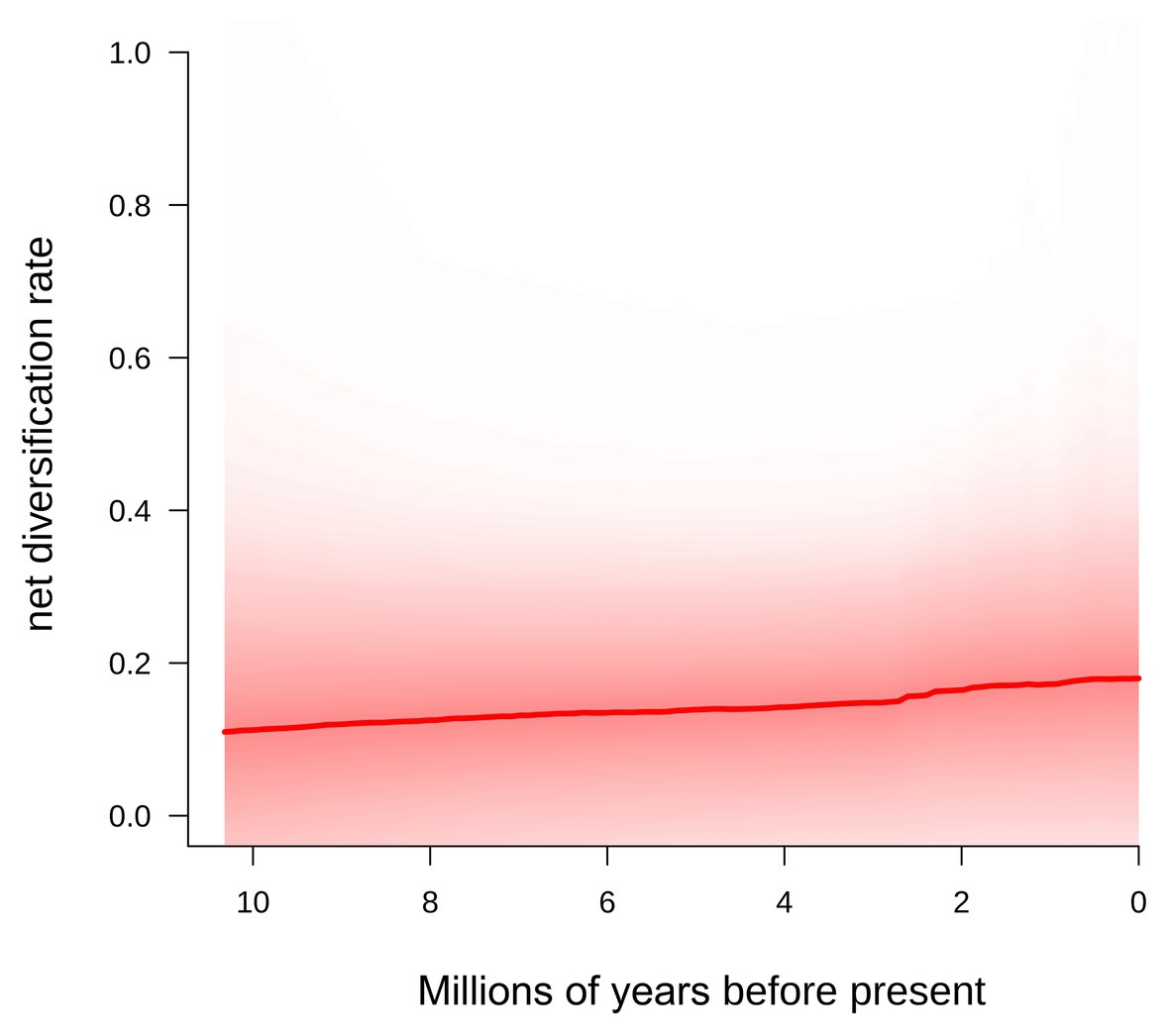
Figure 4. Diversification rate from the origin of the genus Centaurium to the present obtained using BAMM (Rabosky et al., 2013, 2014a; Shi and Rabosky, 2015).
Chromosomal Evolution and Biogeography
The best-fitting model for chromosome evolution based on AIC values was “BASE_NUM_DUPL” (AIC = 116.989; see Supplementary Table 2 for AIC values of all models tested), which considered that chromosome changes were driven by the gain or loss of a chromosome pair, whole-genome duplication (polyploidization) or changes in the basic number (Glick and Mayrose, 2014). This scenario of chromosome evolution showed a rate of 0.52 loss events/Myr, and a gain of 0.00 events/Myr. The rate for duplication of the chromosome number was 0.81 duplications/Myr. For the MRCA of Centaurium, the estimated chromosome number was n = 10 (Figure 1). This is expected to be the ancestral chromosome number for the genus. Our results showed how polyploidization occurred mainly in the tips of the phylogeny in the case of the large western clade (only one polyploidization event inferred in the very shallow node of the subspecies Centaurium erythraea ssp. erythraea and ssp. rhodense), whereas in the widespread clade, there is one ancestral polyploidization event reconstructed at the MRCA node of the species C. mairei, C. capense, and C. centauroides. Specifically, a chromosome loss event was predicted for the MRCA of the clade composed of C. capense, C. centaurioides, C. mairei, and C. pulchellum (base number shifts from 10 to 9), and a later polyploidization event in the case of the MRCA of C. capense, C. centaurioides, and C. mairei.
The analyses using Pagel’s method retrieved dependent correlated evolution between polyploidy and geography, where polyploidy depended on the area where the species and subspecies occurs (Figure 5). Initially, the dispersal rate was independent of polyploidy (from the area of origin to new areas 0.410 events/Myr and vice-versa, 0.599 events/Myr). Second, whereas transitions from diploidy to polyploidy were almost null in the area of origin (0.011 events/Myr), the rate of transition from diploidy to polyploidy in species and subspecies inhabiting new areas or with widespread distribution was 0.841 events/Myr.
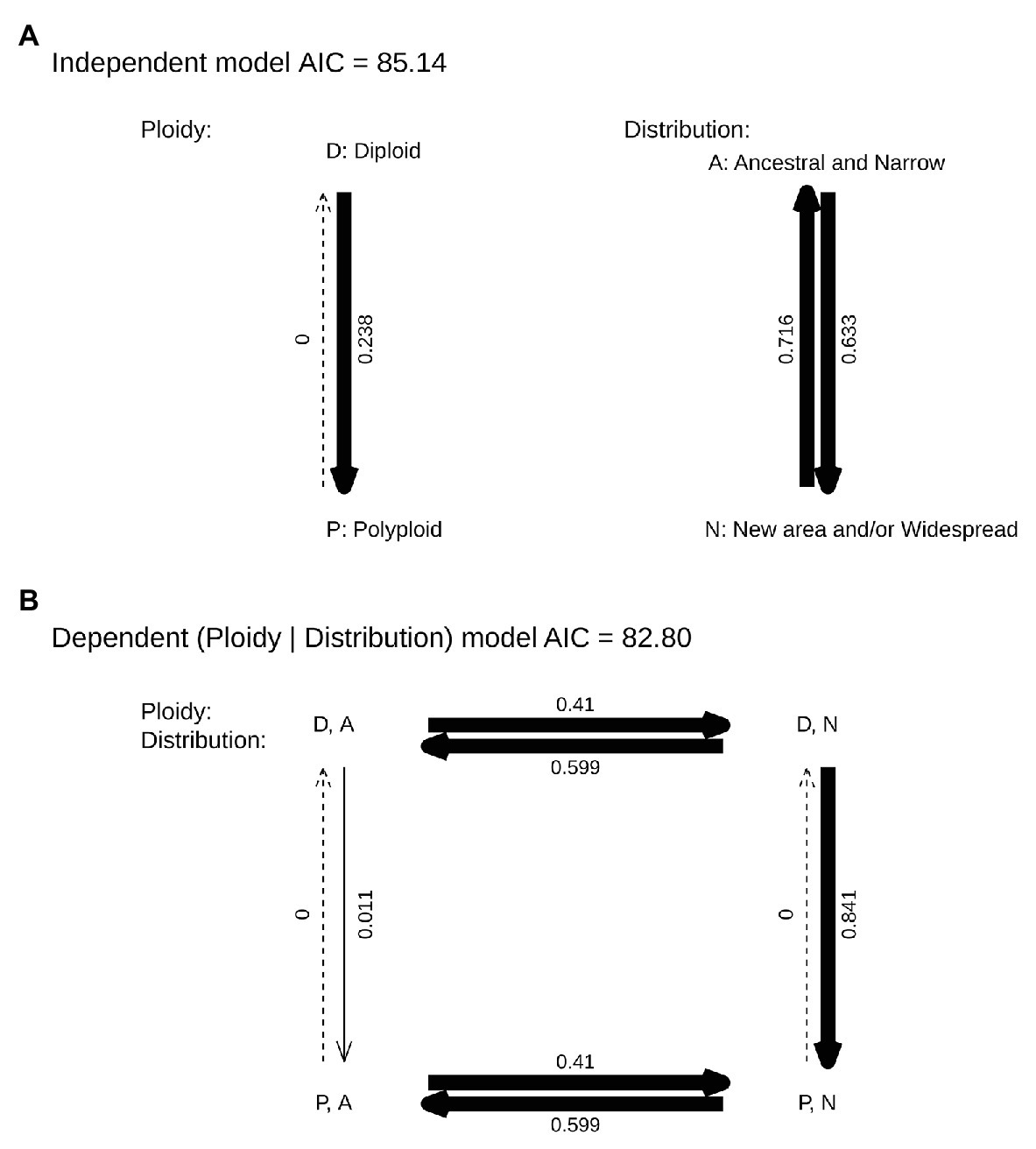
Figure 5. Pagel’s binary correlation test of polyploidy (D for diploid, P for polyploid) and the presence in the area of origin of the species (A if the species is in the ancestral area, N if the species has colonized a new area even if it is still present in its ancestral area and/or have widespread distribution) in Centaurium. Independent (A) and dependent (B) models are shown. Arrow thickness indicates rate proportion.
Discussion
Origin and Evolution of Centaurium
Based on the best fitting model (DECc, AICw = 0.82; Table 1), two main clades – the western and widespread clade – originated from their MRCA. Nevertheless, the best fitting model (regardless of whether founder event “j” was included as a free parameter) could not confidently reconstruct the origin of the MRCA of the whole genus Centaurium (Table 1; Figure 1; Supplementary Figure 1), originated during the Miocene (Jiménez-Lobato et al., 2019). The western clade that originated most likely in the western Mediterranean, which includes almost 70% of extant taxa in the genus (Figure 1), started diversifying about 8.1 Ma (Jiménez-Lobato et al., 2019). Most taxa in this clade occur in the western Mediterranean, only one species with two subspecies (Centaurium littorale) is not present in the Mediterranean but only in northern Europe. The other clade (with ca. 30% of extant taxa) has up to 40% of the species and subspecies with widespread ranges and combining different distribution patterns including the six coding areas in our analyses. For example, C. pulchellum is present in all of the codified areas (but North America), C. mairei in the eastern Mediterranean, Africa, and west and central Asia, and C. maritimum in the whole Mediterranean region, whereas other species have more restricted distribution; for example, C. capense is found in North America and C. centaurioides and C. malzacianum in west and central Asia, and C. discolor in the western Mediterranean (Figure 1). This complex and wide range of species occurrences made BioGeoBEARS unable to reconstruct the geographic origin of deeper nodes in this clade, leaving the biogeographic history of these species partially unknown. The ancestral distribution of the widespread clade was inferred as a broad distribution including west and central Asia but the specific composition of this inferred broad ancestral distribution is very uncertain.
In Centaurium, as in many other groups of plants, the Mediterranean basin acted as a source for later colonization of adjacent areas after glaciations (Thompson, 2020). The origin of most dispersal events in the genus was the western Mediterranean (Figure 3). The most common destinations were adjacent areas, such as the eastern Mediterranean and northern Europe when the origin was the western Mediterranean (with a mean of 3.64 and 3.46 dispersal events, respectively; Figure 3), and the western Mediterranean and west and central Asia in the case of dispersal from the eastern Mediterranean (a mean of 1.16 and 1.84 events, respectively; Figure 3). Interestingly, most dispersal events occurred in recent times in the phylogeny, and all species from the Mediterranean dispersed northward and eastward. Thus, the colonization of southern latitudes, i.e., northern Africa, occurred mainly from western and central Asia and not from the western Mediterranean (Figure 3). Regarding the colonization of North America, the MRCA of C. capense and C. centaurioides presumably dispersed from Asia to North America over the Bering Land Bridge (BLB), when this was open during glacial periods and later diversified into two species: C. capense from North America, and C. centaurioides in west and central Asia, probably during the past million years (Figures 1, 3). The BLB connected Asia and North America during periods where the sea level descended as a consequence of ice formation during glacial periods (Hopkins, 1972; Brikiatis, 2014). The formation of the BLB occurred alternatively during glacial and interglacial periods since the Pliocene (Harris, 2005) and was used by many plant species as a route of dispersal from one continent to the other (e.g., Harris, 2007; Waltari et al., 2007; Mao et al., 2010; Maguilla et al., 2018; among others). The crown node of the separation between C. capense and C. centaurioides was dated by Jiménez-Lobato et al. (2019) sometime during the Pleistocene. This coincides with a period where the BLB was successively closed and then Asia and North America separated (Harris, 2007; Figure 2), supporting the role of the Bering Strait and, more particularly, the alternate opening and closure of the BLB as a dispersal and speciation driver in this clade (Figure 1).
Thus, the importance of the Mediterranean basin for Centaurium lies not only in the fact that it is the place of origin of the MRCA of 70% of the genus (Figure 1), but also as a region where lineages diversified, showing a constant increase in diversification rates from the origin to the present (Figure 4). Presumably, the role of some parts of the Mediterranean as glacial refugia (Petit et al., 2003) allowed diversification of the genus in the region, and were the origins for later dispersals, mainly to adjacent areas during interglacial periods, where more favorable niches were available in upper latitudes.
Polyploidy as a Key to Expand the Range
Diversification in Centaurium was dominated by multiple events of anagenetic dispersals, followed by within-area speciation, then less frequent subset within-area speciation (this is, speciation within part of the distribution of the ancestor but not over the whole area) and rarely, vicariance, as commented above (Figure 2). According to biogeographic reconstruction (Figure 1), most within-area speciation occurred in the western Mediterranean, where most of the species and subspecies in the genus originated. Later, lineages diversified at a constantly increasing rate from the origin to the tips of the phylogeny (Figure 4), while at the same time dispersing, mainly into adjacent areas (Figure 3). When a plant species disperses or colonizes a new area or niche, the success of the event depends on multiple traits. High phenotypic plasticity, capacity for sexual reproduction, high competitiveness with other species or wide range of climatic tolerance are only a few examples of factors affecting the success of establishment in a new area (Baker, 1965; Levin, 2000; Maguilla et al., under review). Polyploidization has been described to have important evolutionary consequences and correlate strongly with range expansion in plants (Levin, 1983; Hijmans et al., 2007; Treier et al., 2009; Te Beest et al., 2012; Soltis et al., 2015). In the Mediterranean region, polyploidy is common among plants, and polyploidization has been demonstrated to be an important trait for diversification in species’ lineages within the region (Vilatersana et al., 2000; Escudero et al., 2018; Thompson, 2020), although it can either increase or decrease rates of diversification (Escudero et al., 2018).
Cytogenetic studies reported two basic chromosome numbers for Centaurium (x = 9 and x = 10; Zeltner, 1970) and during the evolutionary history of the genus, chromosome numbers remained invariable at deeper nodes from an ancestral chromosome number of n = 10 (Figure 1). In the western clade, with most species and subspecies occurring in the area of origin, polyploidization events took place at the tips (with only one exception in a very shallow clade that contains two subspecies), whereas in the widespread clade, with up to ca. 40% of species broadly distributed and inferred broad ancestral areas, a polyploidization event has been reconstructed to be older, as reflected in Figure 1. It is interesting that the widespread clade where up to 40% of species are broadly distributed is also the clade where the percentage of polyploidization events is highest and events deeper in our chromosome number reconstruction (Figure 1). Whereas in the western clade the percentage of polyploids is ca. 50% (mostly tetraploids), the percentage of polyploids in the widespread clade rises to 70% (mostly hexaploids that curiously mostly avoid the Mediterranean region with a clear preference for more arid regions). This widespread clade includes for example C. pulchellum, with a widespread distribution across the northern hemisphere, occurring in all five areas codified for our analyses but North America (Figure 1). Except for C. maritimum from the western and eastern Mediterranean, all other species in this clade (i.e., C. capense, C. centaurioides, C. discolor, C. mairei, C. malzacianum, and C. pulchellum) show some level of polyploidization (Figure 1).
When analyzing the possible correlation between polyploidy and distribution, we found a strong dependence of polyploidy on distribution (Figure 5). Thus, our results confirmed that polyploidy occurred in species and subspecies that are widespread or dispersed out of the area of origin, and suggest that it may have facilitated their establishment and success in the newly colonized area. Thus, polyploidization does not seem to facilitate the dispersal event per se, but rather success in the new or expanded area (Figure 5). Additionally, Mansion et al. (2005) represented geographically the ploidy level of Centaurium species in the Mediterranean basin showing that tetraploids dominated upper latitudes, whereas hexaploids are more common in southern latitudes and diploids in the core of the Mediterranean basin. This is congruent with polyploidy favoring adaptation to different niches or new areas.
Conclusion
We demonstrated how Centaurium species in the western clade remaining in their ancestral area, the western Mediterranean, are diploids. Both recent and ancestral polyploidization events have facilitated the expansion of the distribution range and/or the colonization of new areas outside the areas of origin. Interestingly, Centaurium tetraploid species were more successful colonizing north of the Mediterranean in a more temperate climatic regime. Hexaploid species seem to be more successful growing in southern places in a more arid climatic regime (although our results are not conclusive on whether or not the current range of the hexaploid species is ancestral or recently colonized).
Data Availability Statement
The original contributions presented in the study are included in the article/Supplementary Material, further inquiries can be directed to the corresponding author.
Author Contributions
JA and ME conceived the research topic. CA-C, VJ-L, and ZD-L gathered chromosome data and helped assessing plant taxonomy and distributions. EM and ME performed the analyses. EM wrote the manuscript. All authors contributed to the article and approved the submitted version.
Funding
Project (PGC2018-099608-B-100) funded by: FEDER/Ministerio de Ciencia e Innovación – Agencia Estatal de Investigación. The present work was funded by the Spanish Government and FEDER funds (European Commission) through granted projects CGL2013-45037-P and PGC2018-099608-B-100 to JA, ME, CA-C, EM, and ZD-L, and fellowship FJCI-2017-32314 (“Juan de la Cierva – Formación”) to EM granted by the Spanish Government.
Conflict of Interest
The authors declare that the research was conducted in the absence of any commercial or financial relationships that could be construed as a potential conflict of interest.
Acknowledgments
We are grateful to and dedicated this manuscript to Dr. L. Zeltner for sharing cytogenetic information about the genus Centaurium, which was useful for clarifying the ploidy level of the species. We thank B. M. Rojas-Andrés and V. Valcarcel as reviewers, and associate editor A. A. Crowl for their valuable comments improving the first versions of this manuscript. General Research Services at Universidad de Sevilla (CITIUS2, herbarium and DNA lab), for allowing lab work, and CICA (Scientific Andalusian Informatics Center) for providing computational resources for the analyses.
Supplementary Material
The Supplementary Material for this article can be found online at: https://www.frontiersin.org/articles/10.3389/fpls.2021.650551/full#supplementary-material
References
Affenzeller, M., Kadereit, J. W., and Comes, H. P. (2018). Parallel bursts of recent and rapid radiation in the Mediterranean and Eritreo-Arabian biodiversity hotspots as revealed by Globularia and Campylanthus (Plantaginaceae). J. Biogeogr. 45, 552–566. doi: 10.1111/jbi.13155
Akaike, H. (1974). A new look at the statistical model identification. IEEE Trans. Automat. Contr. 19, 716–7230.
Ali, T., Muñoz-Fuentes, V., Buch, A. K., Çelik, A., Dutbayev, A., Gabrielyan, I., et al. (2019). Out of Transcaucasia: origin of Western and central Palearctic populations of Microthlaspi perfoliatum. Flora Morphol. Distrib. Funct. Ecol. Plants 253, 127–141. doi: 10.1016/j.flora.2019.02.012
Aparicio, A., Escudero, M., Valdés-Florido, A., Pachón, M., Rubio, E., Albaladejo, R. G., et al. (2019). Karyotype evolution in Helianthemum (Cistaceae): Dysploidy, achiasmate meiosis and ecological specialization in H. squamatum, a true gypsophile. Bot. J. Linn. Soc. 191, 484–501. doi: 10.1093/botlinnean/boz066
Avise, J. C. (2000). Phylogeography: The history and formation of species. Cambridge, Massachussets: Harvard Un.
Baker, H. G. (1965). “Characteristics and modes of origin of weeds” in The genetics of colonizing species. eds. H. G. Baker and G. L. Stebbins (NY: Academic Press Inc.), 147–172.
Barres, L., Sanmartín, I., Anderson, C. L., Susanna, A., Buerki, S., Galbany-Casals, M., et al. (2013). Reconstructing the evolution and biogeographic history of tribe Cardueae (compositae). Am. J. Bot. 100, 867–882. doi: 10.3732/ajb.1200058
Barrett, S. C. H. (2002). The evolution of plant sexual diversity. Nat. Rev. Genet. 3, 274–284. doi: 10.1038/nrg776
Bouckaert, R., Heled, J., Kühnert, D., Vaughan, T., Wu, C. H., Xie, D., et al. (2014). BEAST 2: a software platform for bayesian evolutionary analysis. PLoS Comput. Biol. 10, 1–6. doi: 10.1371/journal.pcbi.1003537
Brikiatis, L. (2014). The de geer, thulean and beringia routes: key concepts for understanding early cenozoic biogeography. J. Biogeogr. 41, 1036–1054. doi: 10.1111/jbi.12310
Buerki, S., Jose, S., Yadav, S. R., Goldblatt, P., Manning, J. C., and Forest, F. (2012). Contrasting biogeographic and diversification patterns in two mediterranean-type ecosystems. PLoS One 7:e39377. doi: 10.1371/journal.pone.0039377
Díaz-Lifante, Z. (2012). “Centaurium” in Flora Iberica 11. eds. C. Romero and A. Quintanar (Madrid: Real Jardín Botánico, CSIC), 49–81.
Duffy, K. J., and Johnson, S. D. (2017). Specialized mutualisms may constrain the geographical distribution of flowering plants. Proc. R. Soc. B Biol. Sci. 284:20171841. doi: 10.1098/rspb.2017.1841
Duggen, S., Hoernie, K., Van den Bogaard, P., Rüpke, L., and Morgan, J. P. (2003). Deep roots of the Messinian salinity crisis. Nature 422, 602–606. doi: 10.1038/nature01553
Escudero, M., Balao, F., Martín-Bravo, S., Valente, L., and Valcárcel, V. (2018). Is the diversification of Mediterranean Basin plant lineages coupled to karyotypic changes? Plant Biol. 20, 166–175. doi: 10.1111/plb.12563
Escudero, M., Martín-Bravo, S., Mayrose, I., Fernández-Mazuecos, M., Fiz-Palacios, O., Hipp, A. L., et al. (2014). Karyotypic changes through dysploidy persist longer over evolutionary time than polyploid changes. PLoS One 9:e85266. doi: 10.1371/journal.pone.0085266
Escudero, M., and Wendel, J. F. (2020). The grand sweep of chromosomal evolution in angiosperms. New Phytol. 228, 805–808. doi: 10.1111/nph.16802
Fitzjohn, R. G. (2010). Quantitative traits and diversification. Syst. Biol. 59, 619–633. doi: 10.1093/sysbio/syq053
Fordham, D. A., Brown, S. C., Wigley, T. M. L., and Rahbek, C. (2019). Cradles of diversity are unlikely relics of regional climate stability. Curr. Biol. 29, R356–R357. doi: 10.1016/j.cub.2019.04.001
Glick, L., and Mayrose, I. (2014). ChromEvol: assessing the pattern of chromosome number evolution and the inference of polyploidy along a phylogeny. Mol. Biol. Evol. 31, 1914–1922. doi: 10.1093/molbev/msu122
Guggisberg, A., Bretagnolle, F., and Mansion, G. (2006). Allopolyploid origin of the Mediterranean endemic, Centaurium bianoris (Gentianaceae), inferred by molecular markers. Syst. Bot. 31, 368–379. doi: 10.1600/036364406777585937
Han, T. S., Zheng, Q. J., Onstein, R. E., Rojas-Andrés, B. M., Hauenschild, F., Muellner-Riehl, A. N., et al. (2020). Polyploidy promotes species diversification of Allium through ecological shifts. New Phytol. 225, 571–583. doi: 10.1111/nph.16098
Harris, S. A. (2005). Thermal history of the Arctic Ocean environs adjacent to North America during the last 3.5 Ma and a possible mechanism for the cause of the cold events (major glaciations and permafrost events). Prog. Phys. Geogr. 29, 218–238. doi: 10.1191/0309133305pp444ra
Harris, S. A. (2007). Biodiversity of the alpine vascular flora of the N.W. north American cordillera: the evidence from phyto-geography. Erdkunde 61, 344–356. doi: 10.3112/erdkunde.2007.04.05
Hewitt, G. M. (2004). Genetic consequences of climatic oscillations in the quaternary. Philos. Trans. R. Soc. B Biol. Sci. 359, 183–195. doi: 10.1098/rstb.2003.1388
Hijmans, R. J., Gavrilenko, T., Stephenson, S., Bamberg, J., Salas, A., and Spooner, D. M. (2007). Geographical and environmental range expansion through polyploidy in wild potatoes (Solanum section Petota). Glob. Ecol. Biogeogr. 16, 485–495. doi: 10.1111/j.1466-8238.2007.00308.x
Hilpold, A., Vilatersana, R., Susanna, A., Meseguer, A. S., Boršić, I., Constantinidis, T., et al. (2014). Phylogeny of the Centaurea group (Centaurea, Compositae) - geography is a better predictor than morphology. Mol. Phylogenet. Evol. 77, 195–215. doi: 10.1016/j.ympev.2014.04.022
Hopkins, D. M. (1972). The paleogeography and climatic history of Beringia during late Cenozoic time. Inter-Nord 12, 121–150.
Jiménez-Lobato, V., Escudero, M., Díaz Lifante, Z., Andrés Camacho, C., De Castro, A., Mansion, G., et al. (2019). Evolution of reproductive traits and selfing syndrome in the sub-endemic Mediterranean genus Centaurium Hill (Gentianaceae). Bot. J. Linn. Soc. 191, 216–235. doi: 10.1093/botlinnean/boz036
Krijgsman, W., Hilgen, F. J., Raffi, I., Sierro, F. J., and Wilson, D. S. (1999). Chronology, causes and progression of the Messinian salinity crisis. Nature 400, 652–655. doi: 10.1038/23231
Landis, M., Matzke, N., Mooer, B., and Huelsenbeck, J. (2013). Bayesian analysis of biogeography when the number of areas is large. Syst. Biol. 62, 789–804. doi: 10.1093/sysbio/syt040
Levin, D. A. (1983). Polyploidy and novelty in flowering plants. Am. Nat. 122, 1–25. doi: 10.1086/284115
Levin, D. A. (2000). The origin, expansion, and demise of plant species. Oxford: Oxford Univ. Press.
Maguilla, E., Escudero, M., Hipp, A. L., and Luceño, M. (2017). Allopatric speciation despite historical gene flow: divergence and hybridization in Carex furva and C. lucennoiberica (Cyperaceae) inferred from plastid and nuclear RAD-seq data. Mol. Ecol. 26, 5646–5662. doi: 10.1111/mec.14253
Maguilla, E., Escudero, M., and Luceño, M. (2018). Vicariance versus dispersal across Beringian land bridges to explain circumpolar distribution: a case study in plants with high dispersal potential. J. Biogeogr. 45, 771–783. doi: 10.1111/jbi.13157
Mansion, G., and Struwe, L. (2004). Generic delimitation and phylogenetic relationships within the subtribe Chironiinae (Chironieae: Gentianaceae), with special reference to Centaurium: evidence from nrDNA and cpDNA sequences. Mol. Phylogenet. Evol. 32, 951–977. doi: 10.1016/j.ympev.2004.03.016
Mansion, G., Zeltner, L., and Bretagnolle, F. (2005). Phylogenetic patterns and polyploid evolution within the Mediterranean genus Centaurium (Gentianaceae - Chironieae). Taxon 54, 931–950. doi: 10.2307/25065479
Mao, K., Hao, G., Liu, J., Adams, R. P., and Milne, R. I. (2010). Diversification and biogeography of Juniperus (Cupressaceae): variable diversification rates and multiple intercontinental dispersals. New Phytol. 188, 254–272. doi: 10.1111/j.1469-8137.2010.03351.x
Matzke, N. J. (2013). Probabilistic historical biogeography: new models for founder-event speciation, imperfect detection, and fossils allow improved accuracy and model-testing. Front. Biogeogr. 5, 242–248. doi: 10.21425/F55419694
Matzke, N. J. (2014). Model selection in historical biogeography reveals that founder-event speciation is a crucial process in island clades. Syst. Biol. 63, 951–970. doi: 10.1093/sysbio/syu056
Mayrose, I., Barker, M. S., and Otto, S. P. (2010). Probabilistic models of chromosome number evolution and the inference of polyploidy. Syst. Biol. 59, 132–144. doi: 10.1093/sysbio/syp083
Míguez, M., Gehrke, B., Maguilla, E., Jiménez-Mejías, P., and Martín-Bravo, S. (2017). Carex sect. Rhynchocystis (Cyperaceae): a Miocene subtropical relict in the western Palaearctic showing a dispersal derived Rand Flora pattern. J. Biogeogr. 44, 2211–2224. doi: 10.1111/jbi.13027
Myers, N., Mittermeier, R., Mittermeier, C., da Fonseca, G., and Kent, J. (2000). Biodiversity hotspots for conservation priorities. Nature 403, 853–858. doi: 10.1038/35002501
Noroozi, J., Talebi, A., Doostmohammadi, M., Rumpf, S. B., Linder, H. P., and Schneeweiss, G. M. (2018). Hotspots within a global biodiversity hotspot-areas of endemism are associated with high mountain ranges. Sci. Rep. 8, 1–10. doi: 10.1038/s41598-018-28504-9
Pagel, M. (1994). Detecting correlated evolution on phylogenies: a general method for the comparative analysis of discrete characters. Proc. R. Soc. B Biol. Sci. 255, 37–45. doi: 10.1098/rspb.1994.0006
Petit, R. J., Aguinagalde, I., de Beaulieu, J. -L., Bittkau, C., Brewer, S., Cheddadi, R., et al. (2003). Glacial refugia: hotspots but not melting pots of genetic diversity. Science 300, 1563–1565. doi: 10.1126/science.1083264
Plummer, M., Best, N., Cowles, K., and Vines, K. (2006). CODA: convergence diagnosis and output analysis for MCMC. R News 6, 7–11.
Rabosky, D. L., Donnellan, S. C., Grundler, M., and Lovette, I. J. (2014a). Analysis and visualization of complex macroevolutionary dynamics: an example from Australian Scincid lizards. Syst. Biol. 63, 610–627. doi: 10.1093/sysbio/syu025
Rabosky, D. L., Grundler, M., Anderson, C., Title, P., Shi, J. J., Brown, J. W., et al. (2014b). BAMMtools: an R package for the analysis of evolutionary dynamics on phylogenetic trees. Methods Ecol. Evol. 5, 701–707. doi: 10.1111/2041-210X.12199
Rabosky, D. L., Santini, F., Eastman, J., Smith, S. A., Sidlauskas, B., Chang, J., et al. (2013). Rates of speciation and morphological evolution are correlated across the largest vertebrate radiation. Nat. Commun. 4:1958. doi: 10.1038/ncomms2958
R Core Team (2015). R: A language and environment for statistical computing. Vienna, Austria: R Found. Stat. Comput.
Ree, R. H., and Sanmartín, I. (2018). Conceptual and statistical problems with the DEC+J model of founder-event speciation and its comparison with DEC via model selection. J. Biogeogr. 45, 741–749. doi: 10.1111/jbi.13173
Revell, L. J. (2012). phytools: an R package for phylogenetic comparative biology (and other things). Methods Ecol. Evol. 3, 217–223. doi: 10.1111/j.2041-210X.2011.00169.x
Richard, H. R., and Stephen, A. S. (2008). Maximum likelihood inference of geographic range evolution by dispersal, local extinction, and cladogenesis. Syst. Biol. 57, 4–14. doi: 10.1080/10635150701883881
Ronquist, F. (1997). Dispersal-vicariance analysis: a new approach to the quantification of historical biogeography. Syst. Biol. 46, 195–203. doi: 10.1093/sysbio/46.1.195
Rundel, P. W., Arroyo, M. T., Cowling, R. M., Keeley, J. E., Lamont, B. B., and Vargas, P. (2016). Mediterranean biomes: evolution of their vegetation, floras, and climate. Annu. Rev. Ecol. Evol. Syst. 47, 383–407. doi: 10.1146/annurev-ecolsys-121415-032330
Schneeweiss, G. M., Winkler, M., and Schönswetter, P. (2017). Secondary contact after divergence in allopatry explains current lack of ecogeographical isolation in two hybridizing alpine plant species. J. Biogeogr. 44, 2575–2584. doi: 10.1111/jbi.13071
Shi, J. J., and Rabosky, D. L. (2015). Speciation dynamics during the global radiation of extant bats. Evolution 69, 1528–1545. doi: 10.1111/evo.12681
Soltis, P. S., Marchant, D. B., Van de Peer, Y., and Soltis, D. E. (2015). Polyploidy and genome evolution in plants. Curr. Opin. Genet. Dev. 35, 119–125. doi: 10.1016/j.gde.2015.11.003
Stebbins, G. L. (1947). Types of polyploids: their classification and significance. Adv. Genet. 1, 403–429. doi: 10.1016/S0065-2660(08)60490-3
Tate, J. A., Soltis, P. S., and Soltis, D. E. (2005). “Polyploidy in plants” in The evolution of the genome. eds. T. R. Gregory (Elsevier Science & Technology, Academic Press), 371–426.
Te Beest, M., Le Roux, J. J., Richardson, D. M., Brysting, A. K., Suda, J., Kubešová, M., et al. (2012). The more the better? The role of polyploidy in facilitating plant invasions. Ann. Bot. 109, 19–45. doi: 10.1093/aob/mcr277
Thiers, B. (2015). Index Herbariorum: A global directory of public herbaria and associated staff. New York: Botanical Garden’s Virtual Herbarium.
Treier, U. A., Broennimann, O., Normand, S., Guisan, A., Schaffner, U., Steinger, T., et al. (2009). Shift in cytotype frequency and niche space in the invasive plant Centaurea maculosa. Ecology 90, 1366–1377. doi: 10.1890/08-0420.1
Vilatersana, R., Susanna, A., Garcia-Jacas, N., and Garnatje, T. (2000). Karyology, generic delineation and dysploidy in the genera Carduncellus, Carthamus and Phonus (Asteraceae). Bot. J. Linn. Soc. 134, 425–438. doi: 10.1006/bojl.2000.0349
Waltari, E., Hoberg, E. P., Lessa, E. P., and Cook, J. A. (2007). Eastward ho: Phylogeographical perspectives on colonization of hosts and parasites across the Beringian nexus. J. Biogeogr. 34, 561–574. doi: 10.1111/j.1365-2699.2007.01705.x
Wendel, J. F. (2015). The wondrous cycles of polyploidy in plants. Am. J. Bot. 102, 1753–1756. doi: 10.3732/ajb.1500320
Wood, T. E., Takebayashi, N., Barker, M. S., Mayrose, I., Greenspoon, P. B., and Rieseberg, L. H. (2009). The frequency of polyploid speciation in vascular plants. Proc. Natl. Acad. Sci. 106, 13875–13879. doi: 10.1073/pnas.0811575106
Keywords: biogeography, Centaurium, chromosome, diversification, Mediterranean, niche, polyploidy
Citation: Maguilla E, Escudero M, Jiménez-Lobato V, Díaz-Lifante Z, Andrés-Camacho C and Arroyo J (2021) Polyploidy Expands the Range of Centaurium (Gentianaceae). Front. Plant Sci. 12:650551. doi: 10.3389/fpls.2021.650551
Edited by:
Andrew A. Crowl, Duke University, United StatesReviewed by:
Blanca M. Rojas-Andrés, Leipzig University, GermanyVirginia Valcárcel, Autonomous University of Madrid, Spain
Copyright © 2021 Maguilla, Escudero, Jiménez-Lobato, Díaz-Lifante, Andrés-Camacho and Arroyo. This is an open-access article distributed under the terms of the Creative Commons Attribution License (CC BY). The use, distribution or reproduction in other forums is permitted, provided the original author(s) and the copyright owner(s) are credited and that the original publication in this journal is cited, in accordance with accepted academic practice. No use, distribution or reproduction is permitted which does not comply with these terms.
*Correspondence: Marcial Escudero, amesclir@gmail.com