Recolonization Dynamics of Warm Affinity Halophila nipponica in a Temperate Seagrass Meadow With Zostera marina
- 1Department of Biological Sciences, Pusan National University, Busan, South Korea
- 2School of Earth Sciences and Environmental Engineering, Gwangju Institute of Science and Technology, Gwangju, South Korea
Because Halophila nipponica has only recently been reported in the temperate coastal waters of the northwestern Pacific, the recolonization dynamics of this species have not yet been investigated in temperate seagrass meadows. H. nipponica typically occurs in monoculture or in mixed meadows with Zostera marina, the most abundant seagrass species in this region. In this study, un-bordered and bordered gaps (0.5 × 0.5 m) were created in a mixed seagrass meadow of H. nipponica and Z. marina at Namhae Island on the southern coast of Korea to compare recolonization dynamics of the two species. Un-bordered gaps were marked using only a steel stake at each corner, while the margins of the bordered gaps were blocked to a sediment depth of approximately 20 cm using stainless steel blades to prevent penetration of seagrass rhizomes. Shoot densities of Z. marina and H. nipponica were measured in the gaps and in natural reference plots to estimate percent recolonized. In the bordered gaps, a few Z. marina seedlings and H. nipponica fragments were observed during winter, but no shoots of either species survived to the end of experiment. In the un-bordered gaps, the density of H. nipponica increased rapidly, with approximately 60% recovery after 2 months and reaching 85% after 10 months through only asexual reproduction via clonal growth. By contrast, recolonization of Z. marina was much slower than that of H. nipponica, with only approximately 25% recovery after 10 months through vegetative growth and recruitment of a few seedlings. Thus, small fast-growing H. nipponica rapidly recolonized, compared to relatively large slow-growing Z. marina in the small-size gaps. Asexual reproduction was the principle reproductive mechanism for the recolonization of both Z. marina and H. nipponica at the study site. According to our results, gaps created naturally and anthropogenically in mixed seagrass meadows may be primarily recolonized by H. nipponica rather than Z. marina, leading to a change in the seagrass ecosystem structure in Korean coastal waters.
Introduction
Seagrasses are both economically and ecologically important components of coastal and estuarine ecosystems (Hejnowicz et al., 2015; Dewsbury et al., 2016; Unsworth et al., 2019). However, significant losses of seagrass habitats have recently been reported from many parts of the world (Orth et al., 2006a; Waycott et al., 2009; Short et al., 2014). The disappearance of seagrass meadows has occurred at a rate of 110 km2 yr–1 worldwide since 1980, and the rates of decline have recently accelerated (Waycott et al., 2009). These declines are related to both natural and anthropogenic disturbances such as storms, algal blooms, climate change, fishing activity, coastal construction, and reclamation (Rasheed, 2004; Brodersen et al., 2018; Chefaoui et al., 2018). Such disturbances can create small and/or large gaps in seagrass meadows (Paula et al., 2004; Rasheed, 2004; Neckles et al., 2005). In tropical and subtropical coastal waters, considerable attention has been given to the recovery and recolonization of seagrasses after disturbances of varying scales (Meehan and West, 2000; Morris and Virnstein, 2004; Olesen et al., 2004; Paula et al., 2004; Rasheed, 2004; Walker et al., 2006), however, only a few studies have examined the recolonization in mixed seagrass meadows of temperate seagrasses after disturbances.
Seagrasses can reproduce both sexually through seed production and asexually via clonal growth, but the reproductive efforts of these plants vary widely among species (Hemminga and Duarte, 2000). Seagrass meadows are commonly sustained by clonal growth, which is a key reproductive strategy for the recovery and recolonization of disturbed areas (Rasheed, 2004; González-Correa et al., 2005; Boese et al., 2009). However, sexual reproduction through flowering and seedling establishment is also an important process for the maintenance and recolonization of seagrass meadows (Greve et al., 2005; Lee et al., 2007a; Jarvis et al., 2012). Although flowering frequency and seedling survival rate of seagrasses are typically low, rapid recolonization via sexual reproduction has been observed after large-scale disappearances of seagrasses in some geographical regions (Paula et al., 2004; Orth et al., 2006b; Jarvis and Moore, 2010; Kim et al., 2014). In addition, a two-step seagrass recolonization process has been reported in disturbed areas, whereby shoot density increases via sexual reproduction through seedling establishment during the initial phase, followed by the expansion of seagrass meadows via asexual reproduction through lateral shoot production (Plus et al., 2003; Greve et al., 2005; Lee et al., 2007a; Jarvis et al., 2012). The relative contribution of sexual and asexual reproduction to the maintenance and/or recolonization of seagrass meadows may vary among geographical regions, disturbance scale, and species (Cabaço et al., 2012; Kim et al., 2014; Sherman et al., 2018).
Recolonization of gaps in seagrass meadows is governed by growth strategies of surrounding species (Rollon et al., 1999; Olesen et al., 2004; Rasheed, 2004). Small seagrass species, which are characterized by high growth and rhizome elongation rates, generally play a role as early colonizers in the small-scale disturbed area. The species in genus Halophila, Cymodocea, and Halodule, referred to as pioneer species (guerrilla strategy), have lower resistance, but ability to recolonize rapidly through vegetative growth via asexual reproduction in the disturbed areas (Rollon et al., 1999; Olesen et al., 2004; Rasheed, 2004). On the other hand, relatively larger, slow-growing seagrasses such as genus Thalassia and Enhalus are considered as the latest recolonizer, referred to as climax species (phalanx strategy), in the disturbed areas due to slow horizontal extension rates (Rollon et al., 1999; Olesen et al., 2004). In the mixed seagrass meadows, the disturbed areas were firstly recolonized by smaller, fast-growing pioneer species, and then were eventually displaced by larger, slow-growing climax species (Congdon et al., 2019; Furman et al., 2019). Thus, recolonization pattern is greatly influenced by the traits of the adjacent seagrass.
Atmospheric carbon dioxide (CO2) concentrations have risen by 40% since preindustrial levels, and concomitantly, sea surface temperature (SST) has gradually increased (IPCC, 2013). According to the 5th report of the IPCC, global SST has risen by 0.11°C per decade over the last 40 years and is expected to increase by an additional 2.6–4.8°C by 2100 (IPCC, 2013). This warming trend in the world’s oceans could directly and indirectly affect the physiological function, behavior, and life cycle of all resident organisms, leading to changes in body size, phenology, and biotic interactions including competition and predation (Yamano et al., 2011; Doney et al., 2012; Poloczanska et al., 2013). Recently, climate-driven regime shifts of temperate marine ecosystems have been reported around the world; these shifts are characterized by the poleward expansion of the distribution of tropical and/or subtropical marine organisms (Vergés et al., 2014; Hyndes et al., 2016; Wernberg et al., 2016). Seagrasses are important foundation species in coastal waters, and the distributional range expansion and phenological changes of seagrasses have also been observed in many geographical regions (Diaz-Almela et al., 2007; Kim et al., 2009; Virnstein and Hall, 2009; Gorman et al., 2016).
The Korean peninsula is located in the temperate region of the northwestern Pacific Ocean. In the coastal waters of Korea, nine seagrass species, including five Zostera, two Phyllospadix, Ruppia maritima, and Halophila nipponica, have been reported on soft sediments and rock substrata within the intertidal zone to a water depth of about 15 m (Lee et al., 2018). Although species in the genus Halophila are predominantly distributed in subtropical or tropical waters, a H. nipponica meadow was observed on the south coast of Korea in 2007 for the first time (Kim et al., 2009, 2012). Several H. nipponica meadows have since been discovered along the southern coast of Korea, and they typically occur as monocultures or mixed with Z. marina, the most abundant seagrass species in Korea (Kim et al., 2012; Lee et al., 2018). H. nipponica in temperate regions of the Japanese archipelago was first described as a new species in 2006, but has been previously treated as H. ovalis since early 1900s (Kuo et al., 2006). Thus, H. nipponica is considered as endemic species in warm temperate waters of Korea and Japan influenced by the Kuroshio Current (Uchimura et al., 2008; Kim et al., 2009) whereas Z. marina has been considered as a low-temperature adapted seagrass species (Lee et al., 2005, 2007b). Because optimum growth temperature for H. nipponica was approximately 25°C in summer when Z. marina underwent significant thermal stress (Kim et al., 2012) differences in temperature optima may be partly responsible for recolonization pattern of these two species after disturbances. Varying scales of seagrass loss caused by both natural and anthropogenic disturbances are common in seagrass meadows, but the process of recolonization in mixed seagrass meadows containing both warm and cold affinity species is poorly understood in temperate coastal waters. We compared the recolonization dynamics of warm affinity H. nipponica and cold affinity Z. marina in gaps created in mixed seagrass meadows to predict the resilience of these two species to habitat disturbances. We also investigated the relative importance of sexual and asexual reproduction of both seagrass species during recolonization.
Materials and Methods
Study Site
The study site (34°43′40–N, 128°02′07–E) was located at Namhae Island on the southern coast of the Korean peninsula (Figure 1). The tidal regime is semi-diurnal with a maximum tidal range of approximately 3 m during spring tide (Tide Tables for the coast of Korea, Korea Hydrographic and Oceanographic Administration1). Sediments are mainly characterized by fine sand and silts with less than 3% organic content in sediments. Two seagrass species, H. nipponica and Z. marina, exhibit a distinct vertical zonation at the study site. Z. marina is distributed in slightly shallow water (∼1 to 4 m relative to the mean lower low water; MLLW), whereas H. nipponica occurs at water depths of ∼2 to 7 m relative to MLLW. This study was conducted in a mixed seagrass meadow of H. nipponica and Z. marina with a water depth of approximately 2.5–3 m relative to MLLW from September 2010 to July 2011.
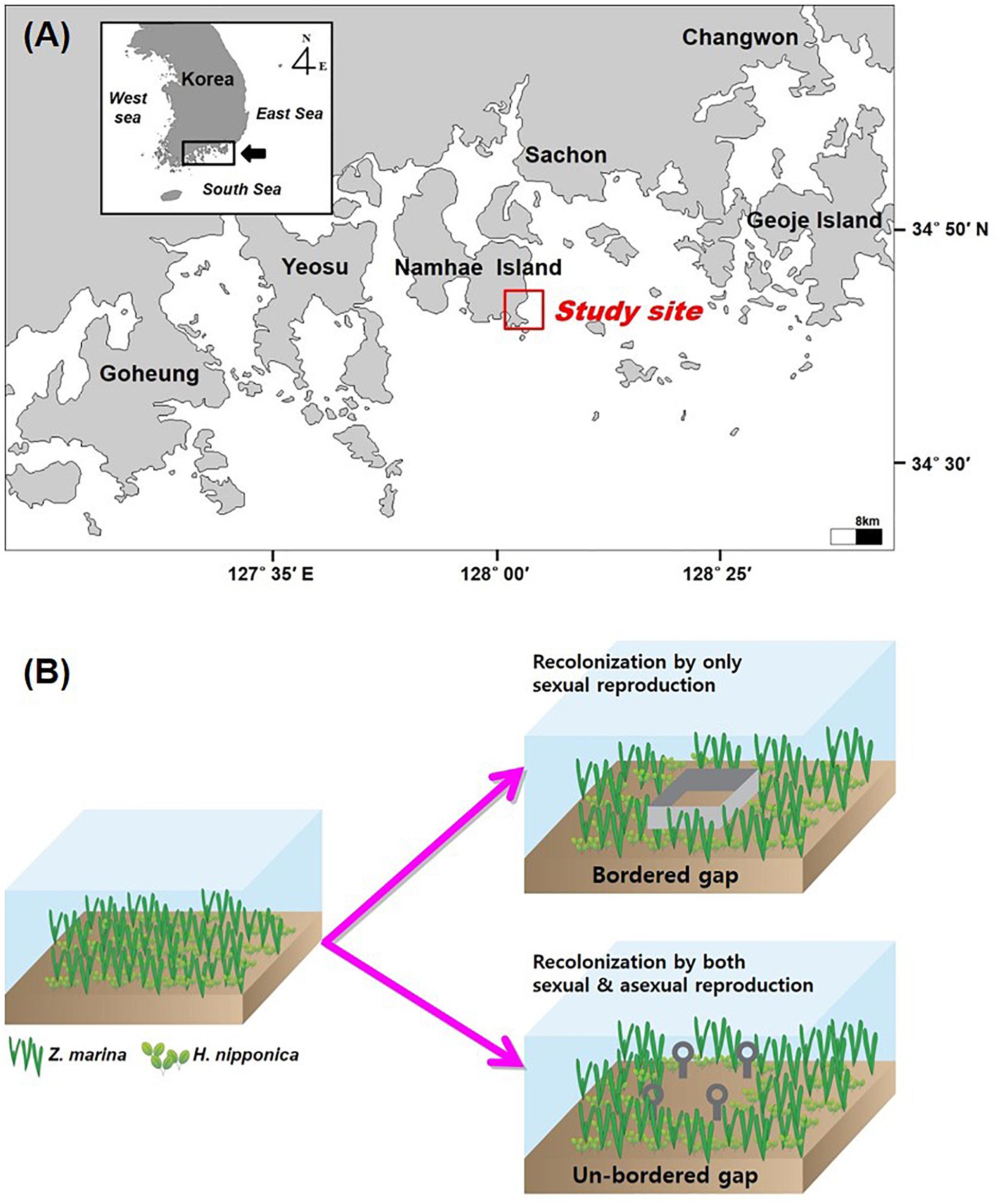
Figure 1. Study site (A) and a diagram of the experimental design for the bordered and un-bordered gaps (B).
Experimental Design
In September 2010, eight experimental gaps (0.5 × 0.5 m) were created in the mixed seagrass meadow at the study site to examine the recolonization of H. nipponica and Z. marina. The seagrass meadows in the study site was close to a small port, and thus the gap size of 0.25 m2 was similar scale to gaps caused by anchoring and boat propeller scarring, which were frequently occurred at the seagrass meadows in this area. Gaps were created by carefully removing all seagrass tissues to minimize sediment loss. Four of the gaps were only marked using a steel stake at each corner (hereafter, the un-bordered gaps), allowing seagrass recolonization by both sexual and asexual reproduction through seedling recruitment and clonal growth, respectively (Figure 1). The margins of the other four gaps were blocked to a sediment depth of approximately 20 cm using stainless steel blades to prevent penetration of seagrass rhizomes (hereafter, the bordered gaps), allowing recolonization by only seedling recruitment (Figure 1). Shoots of H. nipponica and Z. marina in the experimentally created gaps were counted monthly using a grid quadrat (0.5 × 0.5 m) divided into 25 sub-quadrats (0.1 × 0.1 m). Any rhizomes growing over the steel blades of the bordered gaps from the surrounding meadow were removed. Four reference plots were placed at the same tidal level of the experimental gaps to estimate the percent recolonized, which was calculated by dividing shoot density in the gap by average shoot density in the reference plots for each species. All reference plots and experimental gaps were assigned at random. This study was conducted for only 11 months due to a devastating typhoon in August 2011 that destroyed all experimental materials.
Underwater Irradiance and Water Temperature
Underwater photon flux density (PFD) was monitored using an Odyssey photosynthetic irradiance recording system (Dataflow Systems Ltd., Christchurch, New Zealand) every 15 min at the study site from September 2010 to July 2011. The Odyssey sensor was pre-calibrated using an LI-1400 data logger and an LI-193SA spherical quantum sensor (Li-Cor) and was regularly cleaned to minimize fouling. Daily PFD (mol photons m–2 d–1) was calculated as the sum of quantum flux over each 24 h period. In situ water temperature was monitored every 15 min at the study site using StowAway Tidbit temperature data loggers (Onset Computer Corporation, Bourne, MA, United States), and the measured water temperatures were averaged daily.
To detect the warming trend of water temperatures during summer periods on the southern coast of Korea over the last 50 years, water temperature data were obtained from the Korea Oceanographic data center of the National Fisheries Research & Development Institute2, which has monitored water temperature since 1961 at a buoy station, located approximately 40 km from the study site. The yearly number of days with water temperatures exceeding 20°C and 25°C were counted from the 1960 to 2000s.
Statistical Analyses
Values are represented as means ± SEs. Normality and homogeneity of variance were tested for the assumptions of parametric statistics. For each species, a one-way analysis of variance (ANOVA) was used to compare significant differences in shoot density of H. nipponica and Z. marina among reference plots and bordered and un-bordered gaps at the same sampling months. Bordered and un-bordered gaps were separately analyzed due to distinctly different dynamics to test the difference of percent recolonized between two species. Significant difference in percent recolonized between H. nipponica and Z. marina was analyzed using a repeat-measures ANOVA, with the species as the between-subject factor and the month as the within-subject factor. When sphericity assumption was violated, we used Huynh-Feldt epsilon adjusted F values and probabilities. Underwater irradiance and water temperature were each compared among sampling months by one-way ANOVA, with daily total irradiance and average water temperature as samples. If significant differences (α = 0.05) were detected among variables, the Student-Newman-Keuls (SNK) test was performed to determine where significant differences occurred. All statistical analyses were performed using SPSS statistics 20.
Results
Shoot Density
Shoot densities of both H. nipponica and Z. marina in the reference (control) plots exhibited significant seasonal variation, with the highest values (2259 and 185 shoots m–2, respectively), occurring in July 2011 and the lowest values (335 and 107 shoots m–2, respectively), occurring in March 2011 (Figure 2). In the experimentally created gaps, shoot densities of both seagrass species increased more rapidly in the un-bordered gaps than in the bordered gaps (Figure 2). The density of H. nipponica in the un-bordered gaps increased rapidly during the first 2 months of the experiment, after which densities did not significantly differ from those in the reference plots after 9 and 10 months of the experiment (F2,9 = 41.740, p = 0.231 and F2,9 = 73.196, p = 0.104, respectively; Figure 2A). However, in the bordered gaps, H. nipponica did not recolonize via seedling recruitment during the experimental period. The shoot density of Z. marina in the un-bordered gaps increased during the experimental period, but a few seedlings of Z. marina were only observed in the bordered gaps during winter (Figure 2B). In the bordered gaps, a few H. nipponica shoots developed from plant fragments, and seedlings of Z. marina were observed in random locations during winter and spring (Supplementary Figure S1). In the un-bordered gaps, however, both H. nipponica and Z. marina initially recolonized at the periphery of gaps by asexual lateral shoot formation via rhizome branching (Supplementary Figure S2).
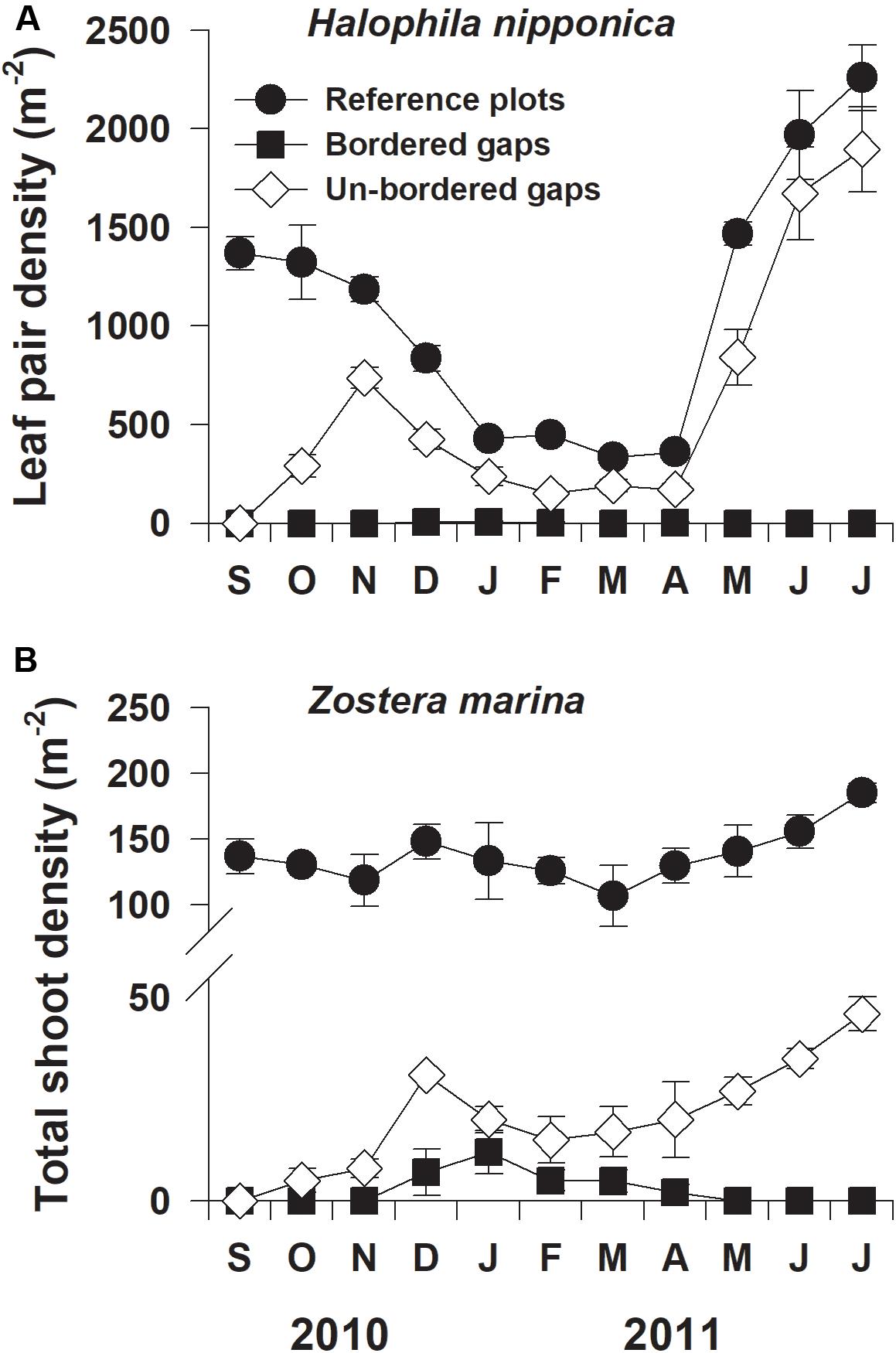
Figure 2. Shoot densities of Halophila nipponica (A) and Zostera marina (B) at the reference plots and the bordered and un-bordered gaps at the study site from September 2010 to July 2011.
Percent Recolonized and Contribution of Asexual and Sexual Reproduction to Recolonization
The percent recolonized of H. nipponica in the un-bordered gaps was significantly (F1,6 = 37.431, p < 0.01) higher than that of Z. marina (Figure 3). In the un-bordered gaps, the percent recolonized of H. nipponica was approximately 60% after 2 months of the experiment, reaching 85% after 10 months (Figure 3A). In the bordered gaps, H. nipponica exhibited a less than 2% of recolonized shoots during winter months (December to February), but no shoots were observed at the end of experiment (Figure 3C). The percent recolonized of Z. marina in the un-bordered gaps increased to approximately 25% after 10 months (Figure 3B). Although a few seedlings of Z. marina were observed in the bordered gaps during winter and spring, no seedlings survived after 8 months of the experiment (Figure 3D).
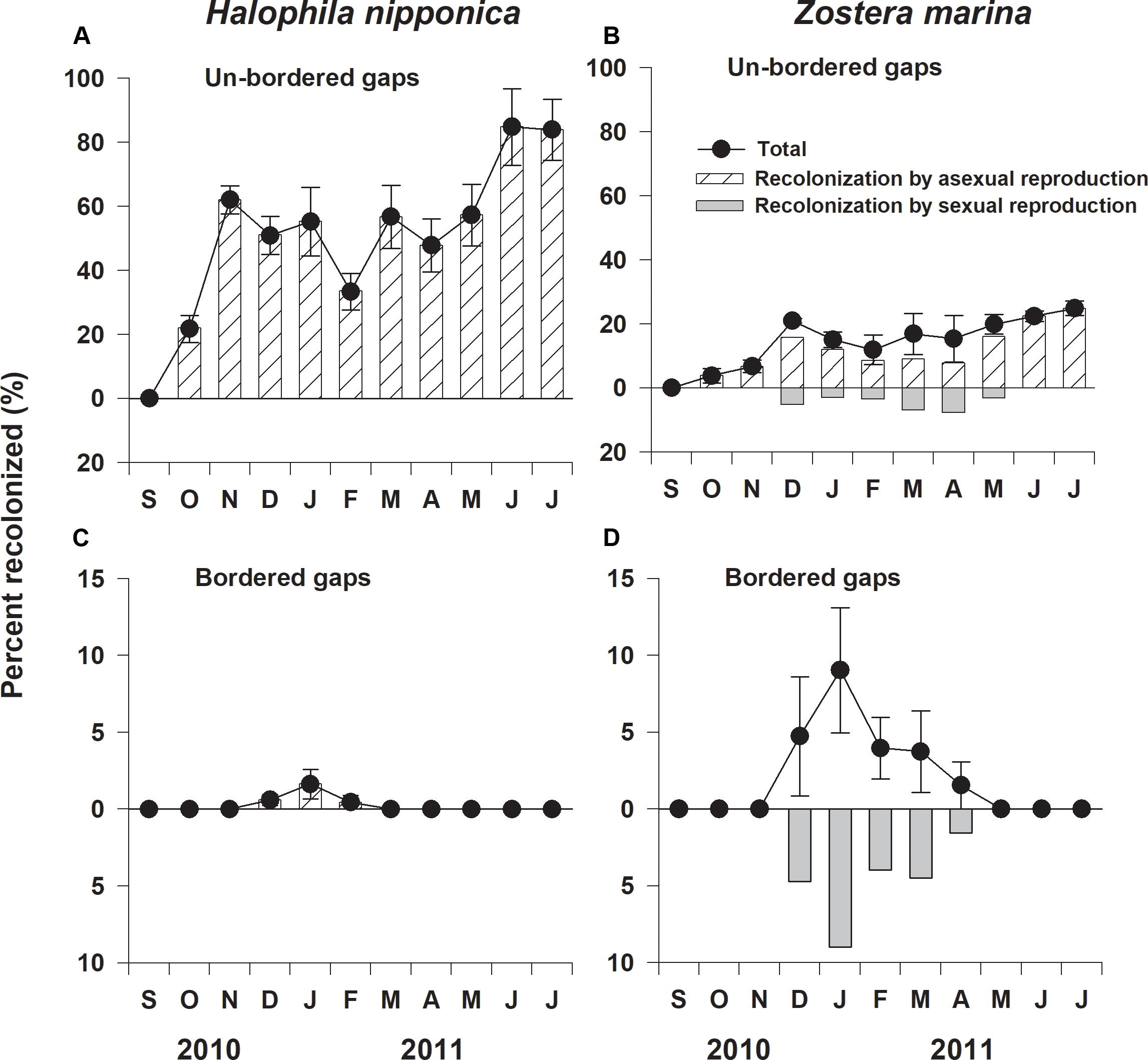
Figure 3. Percent recolonized and relative contribution of sexual and asexual reproduction to recolonization of Halophila nipponica and Zostera marina at the un-bordered (A,B) and bordered (C,D) gaps at the study site from September 2010 to July 2011.
Halophila nipponica only recolonized by asexual reproduction through rhizome branching and vegetative tissue fragment propagules from the adjacent meadow (Figures 3A,C). Although the bordered gaps did not allow rhizome expansion from the adjacent meadow, vegetative tissue fragments of H. nipponica, which developed to new shoots, were observed in the bordered gaps (Figure 3C). Z. marina only recolonized via seedling recruitment during winter and early spring in the bordered gaps, but recolonized by both sexual reproduction via seedling recruitment and asexual reproduction through penetration of rhizomes from the adjacent meadow in the un-bordered gaps (Figures 3B,D). No seedlings of Z. marina successfully established as adult shoots in the experimentally created gaps (Figures 3B,D); thus, the relative contribution of asexual reproduction to the recolonization of Z. marina was much higher than that of sexual reproduction at the study site.
Underwater Irradiance and Water Temperature
Daily underwater irradiance significantly (F10,321 = 7.614, p < 0.001) varied among sampling times (Figure 4A). Monthly average underwater irradiance was highest in July 2011 (11.9 mol photons m–2 d–1) and lowest in February 2011 (3.9 mol photons m–2 d–1), with an average value of 8.1 mol photons m–2 d–1 during the entire experimental period (Figure 4A). Water temperature exhibited clear (F10,322 = 806.797, p < 0.001) seasonal variation, ranging from 7.5°C in mid-February 2011 to 25.9°C in early September 2011 (Figure 4B).
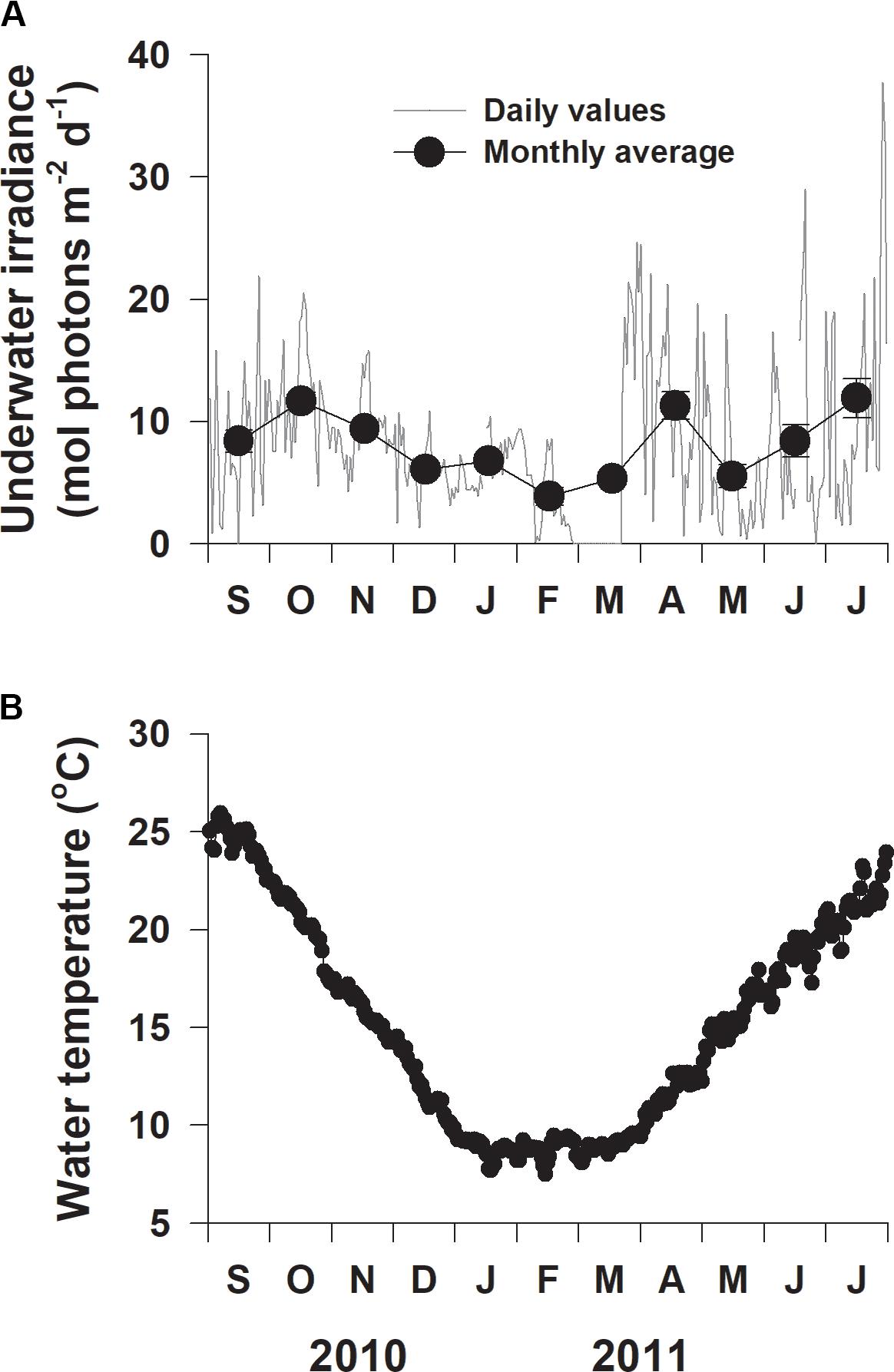
Figure 4. Daily underwater photon flux density (A) and water temperature (B) at the study site on the southern coast of Korea from September 2010 to July 2011.
Summer water temperatures have gradually increased on the southern coast of Korea since the 1960s (Figure 5). The yearly number of days when daily water temperature exceeded 20°C was approximately 109 days during the 1960s, whereas the number of days was approximately 116 days during the 2000s (Figure 5A). The yearly numbers of days when daily water temperature exceeded 25°C were approximately 10 days during the 1960s and 26 days during the 2000s (Figure 5B).
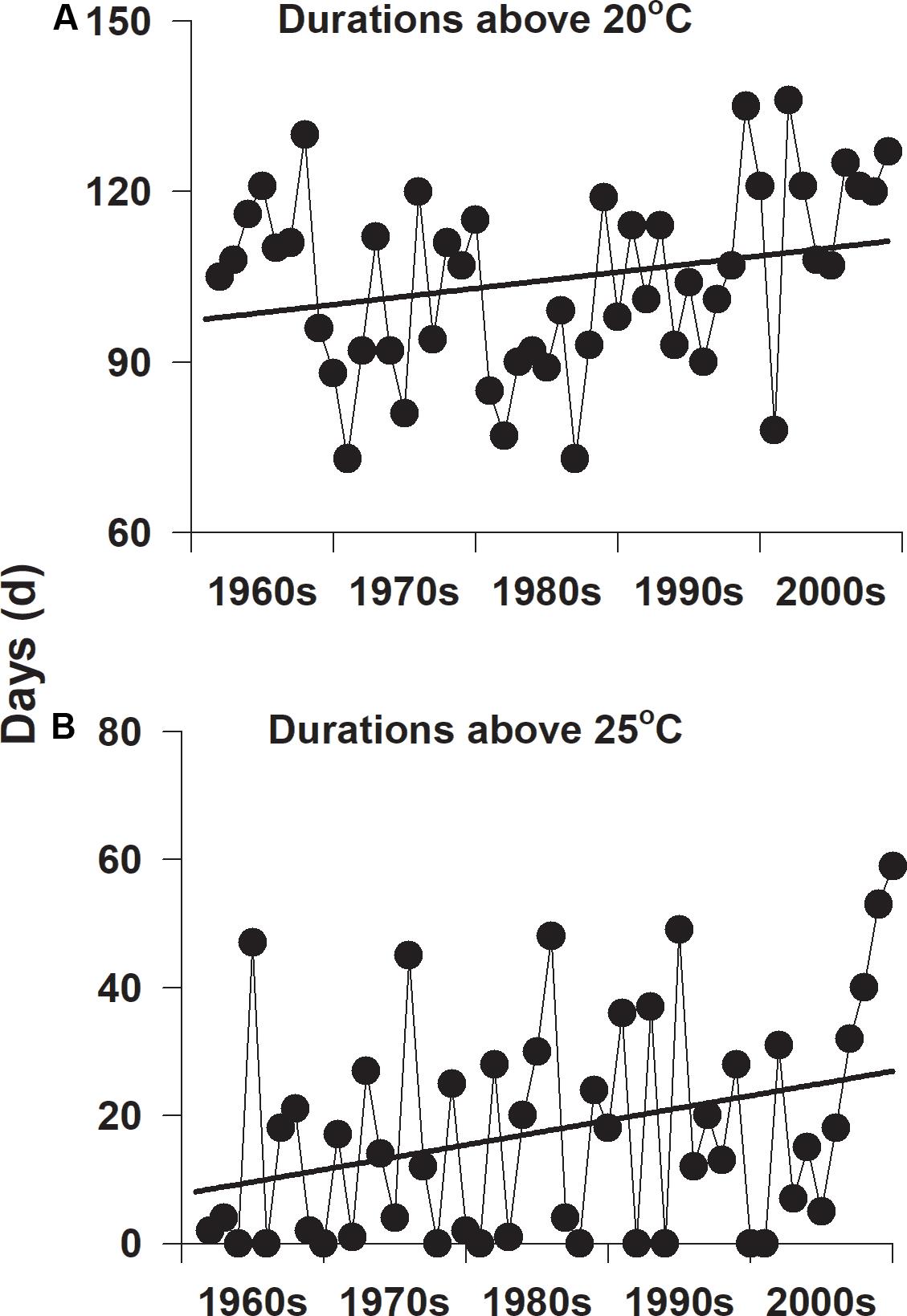
Figure 5. The yearly number of days with temperatures exceeding 20°C (A) and 25°C (B) on the southern coast of Korea since the 1960s.
Discussion
Seagrass Recolonization by Sexual and Asexual Reproduction
The recolonization of seagrasses following destruction caused by various disturbances has been reported in many geographical regions (Morris and Virnstein, 2004; González-Correa et al., 2005; Greve et al., 2005; Orth et al., 2006b; Lee et al., 2007a; Park et al., 2011). Asexual reproduction functions as a primary mechanism for the expansion and recolonization of disturbed seagrass meadows (Olesen et al., 2004; Boese et al., 2009; Park et al., 2011). In a multi-species tropical seagrass meadow, shoot density and above-ground biomass in artificially created gaps recovered to the level of un-manipulated control plots within 10 months through asexual reproduction (Rasheed, 2004). After seagrass loss due to mechanical damage caused by a barge grounding in a shallow reef lagoon in the Philippines, approximately 450 m2 of seagrass vegetation recolonized, and the majority of the net increase in seagrass cover occurred via rhizome penetration from the surrounding seagrass meadow (Olesen et al., 2004). In the present study, although a few seedlings of Z. marina were observed in the gaps, asexual reproduction was the main mechanism for seagrass recolonization within the artificially created gaps in the mixed seagrass meadow of Z. marina and H. nipponica. The recolonization of seagrasses was much faster in the un-bordered gaps than in the bordered gaps. At the end of the experiment, no seagrass shoots had established in the bordered gaps, in which asexual reproduction through penetration of vegetative shoots and roots from the surrounding seagrass meadows was prevented. By contrast, approximately 85% of H. nipponica and 25% of Z. marina recovered through asexual reproduction in the un-bordered gaps, in which recolonization was allowed through both sexual and asexual reproduction.
Sexual reproduction by seeds and seedling establishment is also an essential recolonization mechanism in large-scale disturbed areas (Plus et al., 2003; Greve et al., 2005; Lee et al., 2007b). Previous studies have documented the rapid recolonization of seagrass beds through the germination of seeds in the sediments following intense destruction caused by various disturbances such as algal blooms and anoxic events (Plus et al., 2003; Lee et al., 2007a). In the present study, Z. marina recolonized by both sexual and asexual reproduction during the initial phase of recovery in the experimentally created gaps. Although a few seedlings of Z. marina were observed in both the bordered and un-bordered gaps during winter, no seedlings developed to adult shoots at the study site. Because seagrass seedlings are vulnerable to abiotic and biotic stresses, survival rates of Z. marina seedlings are often very low (Hauxwell et al., 2001; Greve et al., 2005). In the Yaquina River Estuary, a few seedlings of Z. marina were observed in experimentally created gaps, but none survived until summer (Boese et al., 2009). Z. marina seedlings emerging under a seagrass canopy usually exhibit very low survival rates (Marion and Orth, 2010). Less than 5% of Z. marina seedlings from germinated seeds developed to adult shoots at the shallow area in Jindong Bay on the southern coast of Korea (Kim et al., 2014). Thus, the lower contribution of sexual reproduction to the recolonization of Z. marina in the present study can be partially attributed to the generally low survival rates of seedlings. Sexual reproduction of Z. marina can be a potentially important mechanism, as seedlings were observed during the germination period at our study site. However, seedlings of H. nipponica were not observed in the experimentally created gaps throughout the experimental period; thus, sexual reproduction of this warm temperature-adapted seagrass species (Kim et al., 2012) may rarely occur in temperate coastal waters, although fruits containing seeds of this species have been observed in this region (Kim et al., 2009).
Vegetative tissue fragments are often thought to serve as potential propagules for seagrass dispersal, as they can drift, root, and produce new shoots (Hall et al., 2006; Diaz-Almela et al., 2008; Stafford-Bell et al., 2015). Vegetative fragments of Halodule wrightii and Halophila johnsonii exhibited the ability to settle in mesocosm experiments, indicating that seagrass tissue fragments are able to disperse and recruit in new habitats or disturbed areas (Hall et al., 2006). Campbell (2003) also demonstrated that vegetative fragments of Posidonia species can recruit and colonize new areas. In the present study, in the bordered gaps where root and rhizome penetration into gaps was prevented, a few shoots of H. nipponica were observed during winter and early spring; these did not appear to be seedlings arising from seed germination because of their size. Shoots of H. nipponica growing over the borders of the gaps from the adjacent meadow may not be possible, as the growth of this species is severely limited during winter and early spring in this region (Kim et al., 2012). Because the seagrass meadow at the study site was located near a small port, boat grounding, anchor damage, boat propeller scarring, and fishing activity were frequently observed in the meadow. These physical disturbances may result in the production of vegetative tissue fragments of H. nipponica, which can develop to new shoots. Thus, tissue fragments of H. nipponica can likely function as propagules that can disperse and colonize new habitats.
Recolonization of Halophila nipponica and Zostera marina
Small, fast-growing seagrasses such as species of the genera Cymodocea, Halodule, and Halophila are considered pioneer species, which rapidly colonize new habitats and/or recolonize disturbed seagrass meadows (Rollon et al., 1999; Olesen et al., 2004; Rasheed, 2004). These species are characterized by fast rhizome elongation rates and a high vegetative propagation capacity; thus, disturbed seagrass meadows are rapidly and efficiently recolonized by these pioneer species (Rollon et al., 1999; Olesen et al., 2004; Rasheed, 2004). Halophila ovalis was the most conspicuous early colonizer among six seagrass species in a mixed tropical seagrass meadow, in which the shoot density of H. ovalis reached up to 30-fold higher than pre-disturbance values within 6 months after disturbance (Rollon et al., 1999). Syringodium isoetifolium was also a rapid asexual colonizer of disturbed areas, in which the shoot density of this species reached the same level of the natural meadow within 2 months following the clearing of shoots (Rasheed, 2004). In the present study, H. nipponica exhibited very rapid recolonization in the experimentally created gaps. H. nipponica achieved approximately 60% recovery within 2 months after shoot clearing. However, the shoot density of H. nipponica in the experimentally created gaps decreased during winter and spring, because growth of this species is severely restricted at water temperatures less than 15°C (Kim et al., 2012). Nonetheless, H. nipponica achieved up to 85% recovery within 10 months after shoot removal in this study. Compared to H. nipponica, Z. marina showed relatively slow recolonization in the gaps and only achieved 25% recovery within 10 months after shoot removal.
Rollon et al. (1999) reported that the seasonal timing of disturbance did not affect recolonization patterns at mixed seagrass meadows in tropical waters; however, seasonality would likely more strongly affect the pattern of seagrass recolonization in temperate regions due to seasonal variability in seagrass growth. The growth of Z. marina is highest at around 15–20°C during spring and then dramatically decreases during high summer water temperature periods (Pastres et al., 2004; Lee et al., 2005; Nejrup and Pedersen, 2008; Park et al., 2009). Both sexual and asexual reproduction of Z. marina are more prevalent during winter and spring compared to summer periods (Kim et al., 2014; Qin et al., 2016). Previous studies have demonstrated that the vegetative shoot density of Z. marina rapidly increases during winter and spring through shoot recruitment via lateral shoot formation, but decreases during summer and fall due to transformation into flowering shoots and lower shoot recruitment rates during these seasons (Kim et al., 2008, 2014). High Z. marina seedling recruitment via seed germination also occurs during winter and early spring in the temperate coastal waters of Korea and China (Kim et al., 2008, 2014; Qin et al., 2016; Xu et al., 2018). However, H. nipponica exhibits a different growth pattern relative to Z. marina along the coasts of this region (Kim et al., 2012; Park et al., 2017). Growth of H. nipponica is severely restricted at water temperatures below 15°C during winter and spring, and the highest growth occurs at the highest water temperature (∼25°C) during the summer period in this region (Kim et al., 2012). The rhizome elongation rate of H. nipponica was approximately 1.4 cm apex–1 day–1 during summer, but much lower (0.1 cm apex–1 day–1) during winter (Kim et al., 2012). Because seasonal growth and reproduction distinctly differ between these two seagrass species in Korean coastal waters, the recolonization dynamics of H. nipponica and Z. marina would also likely exhibit seasonal differences. Z. marina would be expected to show more rapid recolonization during winter and spring when reproduction occurs through lateral shoot production and seedling recruitment is high (Kim et al., 2014; Qin et al., 2016). By contrast, H. nipponica would be expected to exhibit fast recolonization during summer, as it still possesses tropical growth characteristics (Kim et al., 2012; Park et al., 2017).
Strong evidence of increasing sea temperatures has been reported along the coasts of Korea and throughout the global oceans (Kim et al., 2009; IPCC, 2013). Water temperatures on the south coast of Korea have increased gradually over the last seven decades (Kim et al., 2009). The average water temperature during the coldest month has increased by 2°C, and the average annual water temperature has increased by approximately 1°C in coastal waters of Korea since the 1930s (Kim et al., 2009). In addition, the yearly numbers of days with daily water temperatures exceeding 20°C and 25°C have increased on the southern coast of Korea since the 1960s (Figure 4). The frequency and magnitude of catastrophic events, including typhoons and algal blooms, have also tended to increase gradually on the coasts of Korea over the last few decades (Kim K. et al., 2015; Kim Y. K. et al., 2015). Three consecutive strong typhoons led to the loss of more than 4 km2 of Z. marina meadow in this region (Kim K. et al., 2015). The disappearance of Z. marina due to severe light reduction by a red tide algal bloom has also been reported in coastal waters of Korea (Lee et al., 2007a). These significant losses of Z. marina meadows in this region usually occur during summer when the maximum growth of H. nipponica was observed at the highest water temperature. Thus, the dominance of H. nipponica over Z. marina will likely strengthen in the temperate seagrass meadows in this region. Consequently, the structure of seagrass ecosystems will be altered within the coastal waters of the northwestern Pacific under conditions of ongoing climate change.
Data Availability Statement
The datasets generated for this study are available on request to the corresponding author.
Author Contributions
SK, YK, and K-SL conceived and designed the experiments. SK and YK performed the experiments. SK, HK, and K-SL analyzed the results. All authors contributed to writing the manuscript and approved the submission.
Funding
This research was supported by the Ministry of Oceans and Fisheries, Korea (Project titles: “Long-term changes in structure and function in the marine ecosystems of Korea” and “Development of blue carbon information system and its assessment for management”) and a National Research Foundation of Korea (NRF) grant funded by the Korean Government (MSIT; NRF-2019R1A2C1090641).
Conflict of Interest
The authors declare that the research was conducted in the absence of any commercial or financial relationships that could be construed as a potential conflict of interest.
Acknowledgments
We thank H. J. Song, S. K. Kang, L.-Z. Qin, Z. Sounan, and P. Zhang for many hours of field and lab assistance.
Supplementary Material
The Supplementary Material for this article can be found online at: https://www.frontiersin.org/articles/10.3389/fmars.2020.00500/full#supplementary-material
Footnotes
References
Boese, B. L., Kaldy, J. E., Clinton, P. J., Eldridge, P. M., and Folger, C. L. (2009). Recolonization of intertidal Zostera marina L. (eelgrass) following experimental shoot removal. J. Exp. Mar. Biol. Ecol. 374, 69–77. doi: 10.1016/j.jembe.2009.04.011
Brodersen, M. M., Pantazi, M., Kokkali, A., Panayotidis, P., Gerakaris, V., Maina, I., et al. (2018). Cumulative impacts from multiple human activities on seagrass meadows in eastern Mediterranean waters: the case of Saronikos Gulf (Aegean Sea, Greece). Environ. Sci. Pollut. Res. 25, 26809–26822. doi: 10.1007/s11356-017-0848-7
Cabaço, S., Santos, R., and Sprung, M. (2012). Population dynamics and production of the seagrass Zostera noltii in colonizing versus established meadows. Mar. Ecol. 33, 280–289. doi: 10.1111/j.1439-0485.2011.00494.x
Campbell, M. L. (2003). Recruitment and colonisation of vegetative fragments of Posidonia australis and Posidonia coriacea. Aquat. Bot. 76, 175–184. doi: 10.1016/S0304-3770(03)00016-0
Chefaoui, R. M., Duarte, C. M., and Serrão, E. A. (2018). Dramatic loss of seagrass habitat under projected climate change in the Mediterranean Sea. Glob. Change Biol. 24, 4919–4928. doi: 10.1111/gcb.14401
Congdon, V. M., Bonsell, C., Cuddy, M. R., and Dunton, K. H. (2019). In the wake of a major hurricane: differential effects on early vs. late successional seagrass species. Limnol. Oceanogr. Lett. 4, 155–163. doi: 10.1002/lol2.10112
Dewsbury, B. M., Bhat, M., and Fourqurean, J. W. (2016). A review of seagrass economic valuations: gaps and progress in valuation approaches. Ecosyst. Serv. 18, 68–77. doi: 10.1016/j.ecoser.2016.02.010
Diaz-Almela, E., Marbà, N., Álvarez, E., Santiago, R., Martínez, R., and Duarte, C. M. (2008). Patch dynamics of the Mediterranean seagrass Posidonia oceanica: implications for recolonisation process. Aquat. Bot. 89, 397–403. doi: 10.1016/j.aquabot.2008.04.012
Diaz-Almela, E., Marbà, N., and Duarte, C. M. (2007). Consequences of Mediterranean warming events in seagrass (Posidonia oceanica) flowering records. Glob. Change Biol. 13, 224–235. doi: 10.1111/j.1365-2486.2006.01260.x
Doney, S. C., Ruckelshaus, M., Duffy, J. E., Barry, J. P., Chan, F., English, C. A., et al. (2012). Climate change impacts on marine ecosystems. Annu. Rev. Mar. Sci. 4, 11–37. doi: 10.1146/annurev-marine-041911-111611
Furman, B. T., Merello, M., Shea, C. P., Kenworthy, W. J., and Hall, M. O. (2019). Monitoring of physically restored seagrass meadows reveals a slow rate of recovery for Thalassia testudinum. Restor. Ecol. 27, 421–430. doi: 10.1111/rec.12877
González-Correa, J. M., Bayle, J. T., Sánchez-Lizaso, J. L., Valle, C., Sánchez-Jerez, P., and Ruiz, J. M. (2005). Recovery of deep Posidonia oceanica meadows degraded by trawling. J. Exp. Mar. Biol. Ecol. 320, 65–76. doi: 10.1016/j.jembe.2004.12.032
Gorman, D., Turra, A., Bergstrom, E. R., and Horta, P. A. (2016). Population expansion of a tropical seagrass (Halophila decipiens) in the southwest Atlantic (Brazil). Aquat. Bot. 132, 30–36. doi: 10.1016/j.aquabot.2016.04.002
Greve, T. M., Krause-Jensen, D., Rasmussen, M. B., and Christensen, P. B. (2005). Means of rapid eelgrass (Zostera marina L.) recolonisation in former dieback areas. Aquat. Bot. 82, 143–156. doi: 10.1016/j.aquabot.2005.03.004
Hall, L. M., Hanisak, M. D., and Virnstein, R. W. (2006). Fragments of the seagrasses Halodule wrightii and Halophila johnsonii as potential recruits in Indian River Lagoon, Florida. Mar. Ecol. Prog. Ser. 310, 109–117. doi: 10.3354/meps310109
Hauxwell, J., Cebrián, J., Furlong, C., and Valiela, I. (2001). Macroalgal canopies contribute to eelgrass (Zostera marina) decline in temperate estuarine ecosystems. Ecology 82, 1007–1022. doi: 10.1890/0012-9658(2001)082[1007:mcctez]2.0.co;2
Hejnowicz, A. P., Kennedy, H., Rudd, M. A., and Huxham, M. R. (2015). Harnessing the climate mitigation, conservation and poverty alleviation potential of seagrasses: prospects for developing blue carbon initiatives and payment for ecosystem service programmes. Front. Mar. Sci. 2:32. doi: 10.3389/fmars.2015.00032
Hemminga, M. A., and Duarte, C. M. (2000). Seagrass Ecology. Cambridge, MA: Cambridge University Press.
Hyndes, G. A., Heck, J. K. L., Vergés, A., Harvey, E. S., Kendrick, G. A., Lavery, P. S., et al. (2016). Accelerating tropicalization and the transformation of temperate seagrass meadows. BioScience 66, 938–948. doi: 10.1093/biosci/biw111
IPCC (2013). Climate Change 2013: The Physical Science Basis. Contribution of Working Group I to the Fifth Assessment Report of the Intergovernmental Panel on Climate Change. Cambridge, MA: Cambridge University Press.
Jarvis, J. C., and Moore, K. A. (2010). The role of seedlings and seed bank viability in the recovery of Chesapeake Bay, USA, Zostera marina populations following a large-scale decline. Hydrobiologia 649, 55–68. doi: 10.1007/s10750-010-0258-z
Jarvis, J. C., Moore, K. A., and Kenworthy, W. J. (2012). Characterization and ecological implication of eelgrass life history strategies near the species’ southern limit in the western North Atlantic. Mar. Ecol. Prog. Ser. 444, 43–56. doi: 10.3354/meps09428
Kim, J. B., Park, J.-I., Jung, C.-S., Lee, P.-Y., and Lee, K.-S. (2009). Distributional range extension of the seagrass Halophila nipponica into coastal waters off the Korean peninsula. Aquat. Bot. 90, 269–272. doi: 10.1016/j.aquabot.2008.10.007
Kim, K., Choi, J.-K., Ryu, J.-H., Jeong, H. J., Lee, K., Park, M. G., et al. (2015). Observation of typhoon-induced seagrass die-off using remote sensing. Estuar. Coast. Shelf. S. 154, 111–121. doi: 10.1016/j.ecss.2014.12.036
Kim, S. H., Kim, J. H., Park, S. R., and Lee, K. S. (2014). Annual and perennial life history strategies of Zostera marina populations under different light regimes. Mar. Ecol. Prog. Ser. 509, 1–13. doi: 10.3354/meps10899
Kim, S. H., Kim, Y. K., Park, S. R., Li, W.-T., and Lee, K.-S. (2012). Growth dynamics of the seagrass Halophila nipponica, recently discovered in temperate coastal waters of the Korean peninsula. Mar. Biol. 159, 255–267. doi: 10.1007/s00227-011-1804-6
Kim, T. H., Rark, S. R., Kim, Y. K., Kim, J.-H., Kim, S. H., Kim, J. H., et al. (2008). Growth dynamics and carbon incorporation of the seagrass, Zostera marina L. in Jindong Bay and Gamak Bay on the southern coast of Korea. Algae 23, 241–250. doi: 10.4490/algae.2008.23.3.241
Kim, Y. K., Kim, S. H., and Lee, K.-S. (2015). Seasonal growth responses of the seagrass Zostera marina under severely diminished light conditions. Estuar. Coast. 38, 558–568. doi: 10.1007/s12237-014-9833-2
Kuo, J., Kanamoto, Z., Iizumi, H., and Mukai, H. (2006). Seagrasses of the genus Halophila thouars (Hydrocharitaceae) from Japan. Acta. Phytotax. Geobot. 57, 129–154.
Lee, K.-S., Kim, S. H., and Kim, Y. K. (2018). “Current status of seagrass habitat in Korea,” in The Wetland Book: II: Distribution, Description, and Conservation, eds C. M. Finlayson, G. R. Milton, R. C. Prentice, and N. C. Davidson (Dordrecht: Springer), 1589–1596. doi: 10.1007/978-94-007-4001-3_264
Lee, K.-S., Park, J.-I., Kim, Y. K., Park, S. R., and Kim, J.-H. (2007a). Recolonization of Zostera marina following destruction caused by a red tide algal bloom: the role of new shoot recruitment from seed banks. Mar. Ecol. Prog. Ser. 342, 105–115. doi: 10.3354/meps342105
Lee, K.-S., Park, S. R., and Kim, J.-B. (2005). Production dynamics of the eelgrass, Zostera marina in two bay systems on the south coast of the Korean peninsula. Mar. Biol. 147, 1091–1108. doi: 10.1007/s00227-005-0011-8
Lee, K.-S., Park, S. R., and Kim, Y. K. (2007b). Effects of irradiance, temperature, and nutrients on growth dynamics of seagrasses: a review. J. Exp. Mar. Biol. Ecol. 350, 144–175. doi: 10.1016/j.jembe.2007.06.016
Marion, S. R., and Orth, R. J. (2010). Factors influencing seedling establishment rates in Zostera marina and their implications for seagrass restoration. Restor. Ecol. 18, 549–559. doi: 10.1111/j.1526-100x.2010.00695.x
Meehan, A. J., and West, R. J. (2000). Recovery times for a damaged Posidonia australis bed in south eastern Australia. Aquat. Bot. 67, 161–167. doi: 10.1016/S0304-3770(99)00097-2
Morris, L. J., and Virnstein, R. W. (2004). The demise and recovery of seagrass in the northern Indian River Lagoon, Florida. Estuaries 27, 915–922. doi: 10.1007/bf02803418
Neckles, H. A., Short, F. T., Barker, S., and Kopp, B. S. (2005). Disturbance of eelgrass Zostera marina by commercial mussel Mytilus edulis harvesting in Maine: dragging impacts and habitat recovery. Mar. Ecol. Prog. Ser. 285, 57–73. doi: 10.3354/meps285057
Nejrup, L. B., and Pedersen, M. F. (2008). Effects of salinity and water temperature on the ecological performance of Zostera marina. Aquat. Bot. 88, 239–246. doi: 10.1016/j.aquabot.2007.10.006
Olesen, B., Marba, N., Duarte, C. M., Savela, R. S., and Fortes, M. D. (2004). Recolonization dynamics in a mixed seagrass meadow: the role of clonal versus sexual processes. Estuaries 27, 770–780. doi: 10.1007/bf02912039
Orth, R. J., Carruthers, T. J. B., Dennison, W. C., Duarte, C. M., Fourqurean, J. W., Heck, K. L., et al. (2006a). A global crisis for seagrass ecosystems. BioScience 56, 987–996.
Orth, R. J., Luckenbach, M. L., Marion, S. R., Moore, K. A., and Wilcox, D. J. (2006b). Seagrass recovery in the Delmarva Coastal Bays, USA. Aquat. Bot. 84, 26–36. doi: 10.1016/j.aquabot.2005.07.007
Park, J.-I., Kim, J. B., and Lee, K.-S. (2017). A comparison of growth patterns between non-indigenous Halophila nipponica and the native sympatric Zostera marina on the southern coast of the Korean peninsula. Mar. Ecol. 38:e12452. doi: 10.1111/maec.12452
Park, S. R., Kim, J.-H., Kang, C.-K., An, S., Chung, I. K., Kim, J. H., et al. (2009). Current status and ecological roles of Zostera marina after recovery from large-scale reclamation in the Nakdong River estuary, Korea. Estuar. Coast. Shelf. S. 81, 38–48. doi: 10.1016/j.ecss.2008.10.003
Park, S. R., Kim, Y. K., Kim, J.-H., Kang, C.-K., and Lee, K.-S. (2011). Rapid recovery of the intertidal seagrass Zostera japonica following intense Manila clam (Ruditapes philippinarum) harvesting activity in Korea. J. Exp. Mar. Biol. Ecol. 407, 275–283. doi: 10.1016/j.jembe.2011.06.023
Pastres, R., Brigolin, D., Petrizzo, A., and Zucchetta, M. (2004). Testing the robustness of primary production models in shallow coastal areas: a case study. Ecol. Model. 179, 221–233. doi: 10.1016/j.ecolmodel.2004.06.006
Paula, E. W., Kenworthy, W. J., Michael, J. D., Kamille, K. H., and Manuel, F. M. (2004). Recruitment of Thalassia testudinum seedlings into physically disturbed seagrass beds. Mar. Ecol. Prog. Ser. 267, 121–131. doi: 10.3354/meps267121
Plus, M., Deslous-Paoli, J.-M., and Dagault, F. (2003). Seagrass (Zostera marina L.) bed recolonisation after anoxia-induced full mortality. Aquat. Bot. 77, 121–134. doi: 10.1016/S0304-3770(03)00089-5
Poloczanska, E. S., Brown, C. J., Sydeman, W. J., Kiessling, W., Schoeman, D. S., Moore, P. J., et al. (2013). Global imprint of climate change on marine life. Nat. Clim. Chang. 3:919. doi: 10.1038/nclimate1958
Qin, L.-Z., Li, W.-T., Zhang, X., Zhang, P., and Qiao, W. (2016). Recovery of the eelgrass Zostera marina following intense Manila clam Ruditapes philippinarum harvesting disturbance in China: the role and fate of seedlings. Aquat. Bot. 130, 27–36. doi: 10.1016/j.aquabot.2016.01.002
Rasheed, M. A. (2004). Recovery and succession in a multi-species tropical seagrass meadow following experimental disturbance: the role of sexual and asexual reproduction. J. Exp. Mar. Biol. Ecol. 310, 13–45. doi: 10.1016/j.jembe.2004.03.022
Rollon, R. N., Van Steveninck, E. D. D. R., Van Vierssen, W., and Fortes, M. D. (1999). Contrasting recolonization strategies in multi-species seagrass meadows. Mar. Pollut. Bull. 37, 450–459. doi: 10.1016/S0025-326X(99)00105-8
Sherman, C. D., Smith, T. M., York, P. H., Jarvis, J. C., Ruiz-Montoya, L., and Kendrick, G. A. (2018). “Reproductive, dispersal and recruitment strategies in Australian seagrasses,” in Seagrasses of Australia, eds P. J. Ralph and A. Larkum (Cham: Springer), 213–256. doi: 10.1007/978-3-319-71354-0_8
Short, F. T., Coles, R., Fortes, M. D., Victor, S., Salik, M., Isnain, I., et al. (2014). Monitoring in the Western Pacific region shows evidence of seagrass decline in line with global trends. Mar. Pollut. Bull. 83, 408–416. doi: 10.1016/j.marpolbul.2014.03.036
Stafford-Bell, R. E., Chariton, A. A., and Robinson, R. W. (2015). Prolonged buoyancy and viability of Zostera muelleri Irmisch ex Asch. vegetative fragments indicate a strong dispersal potential. J. Exp. Mar. Biol. Ecol. 464, 52–57. doi: 10.1016/j.jembe.2014.12.014
Uchimura, M., Faye, E. J., Shimada, S., Inoue, T., and Nakamura, Y. (2008). A reassessment of Halophila species (Hydrocharitaceae) diversity with special reference to Japanese representatives. Bot. Mar. 51, 258–268. doi: 10.1515/BOT.2008.036
Unsworth, R. K. K., Nordlund, L. M., and Cullen-Unsworth, L. C. (2019). Seagrass meadows support global fisheries production. Conserv. Lett. 12:e12566. doi: 10.1111/conl.12566
Vergés, A., Steinberg, P. D., Hay, M. E., Poore, A. G. B., Campbell, A. H., Ballesteros, E., et al. (2014). The tropicalization of temperate marine ecosystems: climate-mediated changes in herbivory and community phase shifts. Proc. R. Soc. B Biol. Sci. 281:20140846. doi: 10.1098/rspb.2014.0846
Virnstein, R. W., and Hall, L. M. (2009). Northern range extension of the seagrasses Halophila johnsonii and Halophila decipiens along the east coast of Florida, USA. Aquat. Bot. 90, 89–92. doi: 10.1016/j.aquabot.2008.05.007
Walker, D. I., Kendrick, G. A., and McComb, A. J. (2006). “Decline and recovery of seagrass ecosystems—the dynamics of change,” in Seagrasses: Biology, Ecology and Conservation, eds A. W. D. Larkum, R. J. Orth, and C. M. Duarte (Dordrecht: Springer), 551–565. doi: 10.1007/1-4020-2983-7_23
Waycott, M., Duarte, C. M., Carruthers, T. J. B., Orth, R. J., Dennison, W. C., Olyarnik, S., et al. (2009). Accelerating loss of seagrasses across the globe threatens coastal ecosystems. Proc. Natl. Acad. Sci. U.S.A. 106, 12377–12381. doi: 10.1073/pnas.0905620106
Wernberg, T., Bennett, S., Babcock, R. C., de Bettignies, T., Cure, K., Depczynski, M., et al. (2016). Climate-driven regime shift of a temperate marine ecosystem. Science 353, 169–172. doi: 10.1126/science.aad8745
Xu, S., Wang, P., Zhou, Y., Zhang, X., Gu, R., Liu, X., et al. (2018). New insights into different reproductive effort and sexual recruitment contribution between two geographic Zostera marina L. populations in temperate China. Front. Plant Sci. 9:15. doi: 10.3389/fpls.2018.00015
Keywords: asexual reproduction, climate change, gap, Halophila nipponica, recolonization, Zostera marina
Citation: Kim SH, Kim YK, Kim H and Lee K-S (2020) Recolonization Dynamics of Warm Affinity Halophila nipponica in a Temperate Seagrass Meadow With Zostera marina. Front. Mar. Sci. 7:500. doi: 10.3389/fmars.2020.00500
Received: 20 February 2020; Accepted: 03 June 2020;
Published: 24 June 2020.
Edited by:
Stelios Katsanevakis, University of the Aegean, GreeceReviewed by:
Yi Zhou, Institute of Oceanology (CAS), ChinaJennifer Li Ruesink, University of Washington, United States
Copyright © 2020 Kim, Kim, Kim and Lee. This is an open-access article distributed under the terms of the Creative Commons Attribution License (CC BY). The use, distribution or reproduction in other forums is permitted, provided the original author(s) and the copyright owner(s) are credited and that the original publication in this journal is cited, in accordance with accepted academic practice. No use, distribution or reproduction is permitted which does not comply with these terms.
*Correspondence: Kun-Seop Lee, klee@pusan.ac.kr