Effects of plant diversity and big-sized trees on ecosystem function in a tropical montane evergreen broad-leaved forest
- 1Institute of Highland Forest Science, Chinese Academy of Forestry, Kunming, China
- 2Pu’er Forest Ecosystem Research Station, National Forestry and Grassland Administration of China, Kunming, China
- 3Taiyanghe Provincial Nature Reserve, Pu’er Forestry Bureau, Pu’er, Yunnan, China
Introduction: Scale dependencies play a vital role in defining the biodiversity-ecosystem functioning relationship in forest ecosystems, which varies based on the magnitude of multiple plant diversity attributes, soil properties, and aboveground biomass in forest ecosystems. However, the effects of plant diversity and big-sized trees on the relationship between plant diversity and aboveground biomass across different scales remain unclear in forest ecosystems.
Methods: Based on a 30-ha tropical montane evergreen broad-leaved forest dynamics plot in Yunnan province, China, we comparatively analyzed the importance of scale-dependent effects of multiple plant diversity attributes, soil properties, neighborhood competition intensity and aboveground biomass of big-sized trees, as well as stand structural complexity on aboveground biomass of all woody individuals. The aim is therefore to identify the main predictors for sustaining aboveground biomass of all woody individuals, considering multiple biotic and abiotic factors jointly, as well as underlying mechanisms.
Results: Our results suggest that indicators such as species richness and phylogenetic diversity did not strongly contribute to aboveground biomass of all woody individuals with increasing spatial scales, while aboveground biomass of big-sized trees exhibited the greatest contribution to aboveground biomass of all woody individuals. Stand structural complexity, characterized by variances in woody plant diameter at breast height, also contributed more to aboveground biomass of all woody individuals indirectly via neighborhood competition intensity and aboveground biomass of big-sized trees. Contributions of functional dispersion and community-weighted mean of leaf phosphorus concentration to aboveground biomass of all woody individuals became stronger with increasing spatial scales. Neighborhood competition intensity exhibited a negative linear relationship with aboveground biomass of all woody individuals at the smallest scale, but it affected positively aboveground biomass of all woody individuals across spatial scales, likely due to indirect effects via aboveground biomass of big-sized trees.
Discussion: Big-sized trees will likely become more important in biodiversity maintenance and ecosystem function management as deforestation and forest degradation.
1 Introduction
Recently, investigating the drivers of ecosystem function is one of the most prominent problems in ecological research (Chisholm et al., 2013; van der Sande et al., 2017; Luo et al., 2019; Craven et al., 2020). Many studies have revealed several biotic and abiotic factors that regulate the biodiversity–ecosystem function relationship through underlying mechanisms including sampling effects and complementary effects (Loreau and Hector, 2001; Zhang and Chen, 2015; Grace et al., 2016; Li SF et al., 2018; Luo et al., 2019; Jing et al., 2021). Biodiversity plays several key roles in driving biomass stocks in forest ecosystems (Liang et al., 2016; Liu et al., 2018; Li et al., 2019; Jing et al., 2021; Li et al., 2021). However, species richness only reveals partial mechanisms in driving aboveground biomass stocks in terrestrial ecosystems (Yuan et al., 2019), while multiple biodiversity attributes displayed more important roles including functional dispersion and composition (Finegan et al., 2015; Ali et al., 2017; Yuan et al, 2019; Mahaut et al., 2020), phylogenetic diversity (Flynn et al., 2011; van der Sande et al., 2018; Liang et al., 2019), as well as soil microbial diversity (van der Heijden et al., 2008; Schnitzer et al., 2011; Li et al., 2020a). Recently, more studies have focused on understanding driving mechanisms of stand structural attributes in shaping aboveground biomass in forest ecosystems. Among these, big-sized trees, stand structural complexity, and interspecific interactions are thought to be important drivers the enhancement of ecosystem functioning (Cardinale et al., 2002; Ali, 2019; Ali et al., 2019; Fan et al., 2020; Ali and Wang, 2021).
The ecological role of big-sized trees in forest ecosystem function and service has received increasing attention among ecologists (Mensah et al., 2020; Ali and Wang, 2021). Big-sized trees are now recognized as the main driver in influencing stand-level aboveground biomass in forest ecosystems (Ali et al., 2019; Yuan et al., 2021). Big-sized trees (defined as the top 1% large-diameter trees) have been shown to store up to 50% of biomass stock in temperate forests, exhibiting a greater contribution to biomass was more than that to species diversity (Yuan et al., 2021). However, these large trees are known to be the most susceptible to extreme climatic events and deforestation (McDowell et al., 2020). Big-sized trees generally grow in the overstory and make up the main stand structure, where they exhibit a stronger ability to regulate available resources including light, water, and soil nutrients through niche differentiation (Ali et al., 2019; Yuan et al., 2021), and play an indispensable role in sustaining medium and small trees in the forest. Therefore, studies interested in the biodiversity–ecosystem function relationship of forests should not neglect the role of the “big-sized trees effect” on aboveground biomass, which could provide scientists with a deeper understanding of forest functioning jointly through abiotic and biotic drivers (Ali and Wang, 2021). Variation among individual trees allows for the coexistence of competitors in forest trees (Clark, 2010). Therefore, variation in tree diameters could be considered as stand structural complexity, which could increase forest aboveground biomass through positive plant–plant interactions by the niche complementarity effect (Zhang and Chen, 2015; Ali, 2019).
Local neighborhood interactions also served as a main driver in the biodiversity–productivity relationship (Kunz et al., 2019). Competition for limited resources plays an important role in determining stand dynamics, productivity, and ecological processes (Jucker et al., 2014; Hui et al., 2018). Recently, there has been a growing interest in assessing the effect of competition intensity on shaping ecosystem function in grasslands (Mahaut et al., 2020) and forest ecosystems (Jucker et al., 2014; Zhou et al., 2018). Competition among individual trees displayed significant effects on growth and water use efficiency (De Andrés et al., 2018). Competition intensity could strengthen the complementarity effect of plant diversity on ecosystem functioning in forest ecosystems (Searle and Chen, 2020), which is known to be a crucial factor for driving and regulating biomass allocation (Zhou et al., 2018). In addition, soil nutrients may influence demographic processes at regional or local scales (Zhang and Chen, 2015; van der Sande et al., 2017). For example, in a tropical rainforest, soil nutrient was shown to be more important than plant diversity in driving productivity and biomass stocks (van der Sande et al., 2018). Therefore, it is essential to explore the underlying mechanisms of biotic and abiotic drivers in sustaining ecosystem functioning.
The scale-dependent relationship between biodiversity and ecosystem functioning has been recognized as an important component for providing better guidance for management of biodiversity and ecosystems (Gonzalez et al., 2020). plant species richness changes with spatial scale in a nonlinear fashion due to species composition differences (Chalcraft et al., 2004), with scale-dependence being also the main driver of the phylogenetic structure of tree communities (González-Caro et al., 2021). The scale-dependent relationship between biodiversity and aboveground biomass has received more attention in recent years (Chalcraft et al., 2004; Ni et al., 2007; Chisholm et al., 2013; Fan et al., 2020; Barry et al., 2021). For instance, Chisholm et al. (2013) observed a positive richness–biomass relationship at small scales, and a negative relationship increased with increasing scales in forests. Ni et al. (2007) reported a positive and non-linear relationship in grasslands. However, the effects of spatial scale and plot size on the biodiversity–ecosystem function relationship remains poorly understood (Steur et al., 2020). Specifically, scientists have fewer evidence to jointly assess the scale-dependent effect of multiple diversity attributes, big-sized trees, stand structural complexity, and soil nutrients in driving aboveground biomass of all woody individuals in forest ecosystems.
The tropical montane evergreen broad-leaved forest distributes in tropical mountains of southern Yunnan province, China, with altitudes from 1200 to 1600 m. This forest stores abundant carbon in aboveground biomass (Zhu et al., 2019), and plays a key regulatory role in the regional carbon cycle in southern Yunnan province, China (Li et al., 2020b). Although this forest exhibits extremely rich biodiversity and abundant ecosystem function and service, the biodiversity–ecosystem function relationship for this area still has remains unclear. In an era of increasing climate change and forest degradation, it is essential to assess and predict multiple drivers of forest ecosystem functioning in this forest. In this study, we comparatively analyzed the effects of multiple plant diversity attributes, big-sized trees, stand structural complexity, and soil nutrients on the aboveground biomass of all woody individuals at different scales in a 30-ha forest dynamics plot. We put forward main hypotheses as following: (1) The positive effect of plant diversity on aboveground biomass of all woody individuals decreases with increasing spatial scale; (2) Big-sized trees, rather than stand structural complexity, determine forest aboveground biomass of all woody individuals at different spatial scales; and (3) Neighborhood competition intensity of big-sized trees regulates the relationship between plant diversity and aboveground biomass of all woody individuals with increasing spatial scale. Here, our objectives were to explore the role of plant diversity and big-sized trees in accumulating aboveground biomass, which could provide useful suggestions for sustainable forest ecosystem management in a period of increasing biodiversity loss, deforestation and forest degradation.
2 Materials and methods
2.1 Study area and forest plot design
Our study was conducted in the 30-ha forest dynamics plot of the tropical montane evergreen broad-leaf forest at Taiyanghe Provincial Nature Reserve (22.58°N, 101.13°E). The elevation of the study area ranged from 1462 m to 1580 m. This region has a subtropical monsoon climate with a distinct rainy season from May to October. The average annual temperature and precipitation are 17.7 °C and 1548 mm, respectively (Li et al., 2020b). The soil type of this region is mainly classified as hilly red soil, while the vegetation type is Castanopsis echinocarpa forest, which contains high species richness and complex community structure (Figure 1A). We identified 154,372 individual plants including trees, shrubs, and lianas belonging to 271 species, 178 genera, and 78 families. The dominant species in this plot are Castanopsis echidnoarpa Miq., Lithocarpus fenestratus (Roxb.) Rehd., Schima wallichii (DC.) Choisy, Lithocarpus truncatus (King) Rehd. et Wils., Betula alnoides Buch.-Ham. ex D. Don, Machilus rufipes H. W. Li, Vaccinium exaristatum Kurz, Lindera metcalfiana var. dictyophylla (Allen) H.P.Tsui, Castanopsis calathiformis (Skan) Rehd. et Wils., Litthocarpus grandifolius (D. Don) Biswas, Garuga pierrei Guill., and Ternstroemia gymnanthera (Wight et Arn.) Beddome (Li et al., 2020b). The tropical montane evergreen broad-leaved forest is well-preserved primary forest with few disturbances occurring over 40 years (Li et al., 2020b). These factors make this area an ideal study region for exploring the relationship between biodiversity and ecosystem functioning.
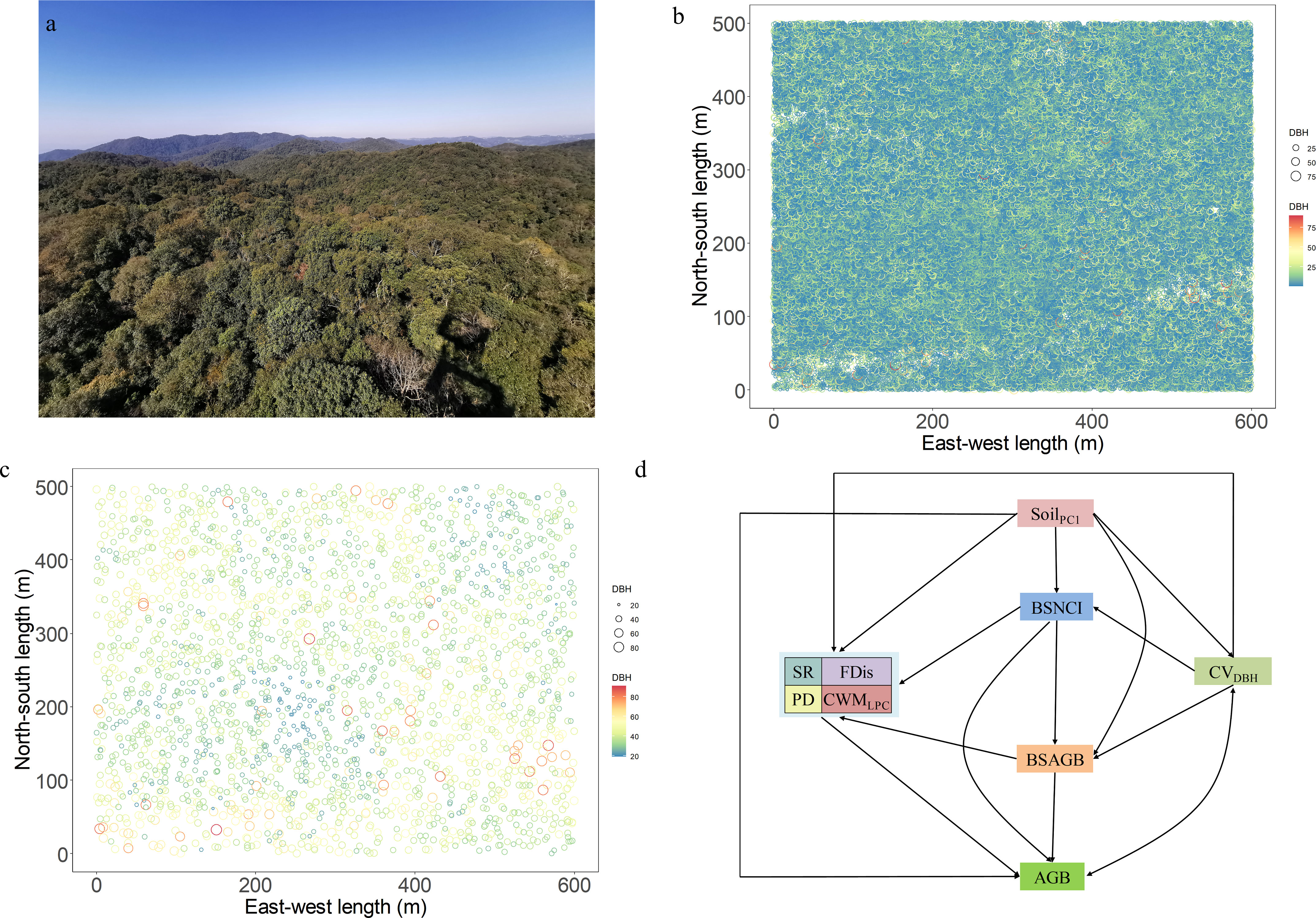
Figure 1 Landscape (A), conceptual framework (B) for the effects of multiple biodiversity attributes, soil nutrients, stand structural complexity (C): spatial distribution of all woody individuals), and big-sized trees (D): spatial distribution) on aboveground biomass of all woody individuals in the 30-ha plot of tropical montane evergreen broad-leaved forest. AGB, aboveground biomass; BSAGB, large tree aboveground biomass; CVDBH, DBH variation; BSNCI, large tree neighborhood competition intensity; SR, species richness; PD, phylogenetic diversity; FDis, functional dispersion; CWMTP, community-weighted mean total phosphorus of leaf; SoilPC1, soil nutrient.
The 30-ha forest dynamics plot (500m×600m) was divided into 750 subplots (20 × 20 m). All living woody individuals with diameter at breast height (DBH≥1cm) were mapped, tagged, measured, and identified in this permanent forest dynamics plot before May 20th, 2019 (Figure 1B). We conducted the following analyses across the plot at five scales: (a) 750 0.04-ha forest plots (20m×20m); (b) 375 0.08-ha forest plots (20m×40m); (c) 187 0.16-ha forest plots (40m×40m); (d) 83 0.36-ha forest plots (60m×60m); and (e) 30 1-ha forest plots (100m×100m).
2.2 Aboveground biomass
We calculated aboveground biomass of all woody individual plants with DBH≥1cm using the general allometric equation based on the DBH (cm), height (H, m), and the species’ wood density (ρ, g•cm−3) as follows (Chave et al., 2014):
Aggregate sums of aboveground biomass was calculated for all woody individuals and big-sized trees, separately, by summing the aboveground biomass measurements at the plot level for each group. Subsequently, the aboveground biomass for five spatial scales was scaled up to t•ha−1.
2.3 Trait sampling and measurement
Five plant functional traits were selected including leaf area, specific leaf area, leaf nitrogen concentration, leaf phosphorus concentration, and wood density. Specific leaf area was chosen as an indicator of light interception efficiency, while leaf nitrogen concentration and phosphorus concentration were selected as indicators of photosynthetic capacity and metabolic rate. In addition, wood density represents volume growth and stem defense (van der Sande et al., 2017; Villa et al., 2020). The community-weighted mean was calculated using the above these trait compositions at the stand level as the sum of the trait values of all species multiplied by their relative basal area. Plant functional traits from 271 woody species were measured, and 10 mature leaves were collected in each woody species. Leaf area was measured using Li-COR 3100C Area Meter (LI-COR, Lincoln, NE, USA). Then, single leaf of each woody species was dried to a constant weight at 65°C for 72 h in the laboratory for leaf dry weight. Specific leaf area was calculated by the ratio of leaf area to dry weight. Next, the leaves of each woody species were composited to measure leaf nitrogen and phosphorus concentrations using a 2400IICHNelemental analyzer (PerkinElmer, Boston, MA, USA) and the molybdenum antimony blue colorimetry, respectively. Wood density of every species was measured using an increment borer (DBH≥5cm) and the water replacement method (DBH<5cm), respectively, which were dried to a constant mass at 65°C for 72 h in the laboratory and weighed using an electronic analytical balance (0.0001g accuracy).
2.4 Soil sampling and physicochemical property analyses
750 soil samples were collected in the 30 hm2 forest dynamic plot at depth of 0~10cm surface soil, which soil composite sample was mixed from three soil samples in each 20m × 20m plot for soil properties including soil pH, soil organic carbon, total nitrogen, total phosphorus, total potassium, hydrolysable nitrogen, available phosphorus, and available potassium were measured. Finally, 750 soil samples were collected in March, 2019, which were sieved through a 2 mm mesh for further analysis. Standard soil property measurement procedures were used to determine soil properties including pH, organic carbon, total nitrogen, total phosphorus, total potassium, hydrolysable nitrogen, available phosphorus, and available potassium. Therein, a pH meter (1:2.5 soil/water ratio) (FE20K, Mettler-Toledo, Greisensee, Switzerland) was to determine soil pH, while a 2400IICHNelemental analyzer (PerkinElmer, Boston, MA, USA) was used to determine soil organic carbon and total nitrogen. Total phosphorus and available phosphorus were determined using the molybdenum antimony blue colorimetry and the Olsen method, while total potassium and available potassium were determined using the atomic absorption photometry method.
2.5 Multiple plant diversity attributes
Species richness was used to quantify the diversity of woody species at each plot, which was calculated as the sum of the total number of woody species with DBH ≥1 cm in the quadrat at five spatial scales. Functional dispersion was calculated using leaf area, specific leaf area, leaf nitrogen concentration, leaf phosphorus concentration, and wood density. Community-weighted means (CWM) of five plant functional traits also were calculated using the average value of traits as well as the relative abundance of species. These analyses were performed using the “FD” package in R 4.4.0 (R Core Team 2021). Faith’s phylogenetic distance was selected to represent phylogenetic diversity (PD) by species relative abundance and phylogenetic distances in each plot (Luo et al., 2019). We calculated Faith’s PD according to data of woody species at DBH≥1cm based on Angiosperm Phylogeny Group (APG) IV. The evolutionary tree was obtained from an online framework (http://phylodiversity.net) using the existing framework. Phyocom software version 4.2 was used to calculate an ultrametric tree with branch lengths scaled to divergent time (Li et al., 2020a).
2.6 Stand structural attributes
Big-sized trees, defined as the 99th percentile for DBH measurements within each plot when all individuals up to a large-diameter threshold in tropical forest were considered (Figure 1C) (Ali et al., 2019). DBH variation was defined as the coefficient of variation in DBH, which was to quantify stand structural complexity using the ratio of the standard deviation to the mean value of DBH of all woody individuals in each plot at five scales.
The neighborhood Hegyi index is a widely used index of conventional competition (Hegyi, 1974), which represents the distance-dependent index. It is calculated using the following equation:
Where Dj and Di are the DBH (cm) of neighboring trees and big-sized tree, respectively, and dij is the horizontal distance (m) between neighboring trees and big-sized tree. n is the number of sample trees. In our study, competition trees are defined as all woody individuals within 6m of a sample tree.
2.7 Data analysis
Variables of interest were characterized using a descriptive statistical analysis via violin plots including aboveground biomass, neighborhood competition intensity, species richness, functional diversity, phylogenetic diversity, DBH variation, CWM, and soil nutrient, which were then natural logarithm transformed for normality and linearity for use in later analysis. All the variables including aboveground biomass of all woody individuals and big-sized trees, neighborhood competition intensity of big-sized trees, species richness, functional diversity, phylogenetic diversity, functional composition, DBH variation as well as soil nutrients were employed to calculate the differences using one-way analysis of variance (ANOVA) at different spatial scales.
To avoid the problem of multicollinearity, we conducted the analysis in two steps. First, a principal component analysis (PCA) on soil properties was used to represent soil nutrients. As the first axis of the PCA (SoilPC1) explained 35.1%, 37.8%, 44.5%, 51.3%, and 56.1% of the total variation across the five scales, respectively, we conclude that SoilPC1 can better represent soil nutrient availability (Figure S1). Second, a Pearson correlation analysis was performed to reduce the bias of high correlation among different CWM, and CWM of leaf phosphorus concentration was used in further analysis due to its > 0.5 correlation coefficient with the other CWM of plant functional traits (Figure S2).
To assess the effects of abiotic and biotic factors on aboveground biomass of all woody individuals, Pearson correlation coefficients firstly were used to measure the pair-wise relationships between aboveground biomass of all woody individuals and each predictor of biodiversity (species richness, functional diversity, phylogenetic diversity, and functional composition), stand structural complexity (DBH variation), big-sized tree attributes (aboveground biomass and neighborhood competition intensity), and soil nutrients at different spatial scales (Figure S3). Bivariate relationships were tested between aboveground biomass of all woody individuals and different response variables using ordinary least squared linear regression across five spatial scales.
To predict the relative contributions of abiotic and biotic factors to aboveground biomass of all woody individuals, a Random Forest analysis was performed to identify the importance of these variables using the package “randomForest” package. Additionally, a causal diagram was utilized to comprehensively test whether the hypothesized abiotic and biotic factors mediate the relationship between multiple biodiversity attributes and ecosystem function using a structural equation model based on a priori knowledge and hypotheses (Rosseel, 2012; Grace et al., 2016; Li SF et al., 2018; Ali et al., 2019; Yuan et al., 2021). Specifically, a prior conceptual framework was used to test the following paths (Figure 1D): (1) species richness, functional dispersion, functional composition, phylogenetic diversity, big-sized tree aboveground biomass and neighborhood competition intensity, stand structural complexity, and soil nutrients directly affect aboveground biomass of all woody individuals; (2) species richness, functional dispersion, functional composition, and phylogenetic diversity indirectly affect aboveground biomass of all woody individuals via aboveground biomass and neighborhood competition intensity of big-sized trees, and stand structural complexity; (3) neighborhood competition intensity of big-sized trees and stand structural complexity indirectly affect aboveground biomass of all woody individuals via aboveground biomass of big-sized trees; and (4) soil nutrients indirectly affect aboveground biomass of all woody individuals via multiple biotic factors. To determine model fitness, metrics including the chi-square test (P>0.05), root square mean errors of approximation (RMSEA<0.05), and goodness-of-fit index (GFI>0.95) were utilized (Rosseel, 2012; Zhang and Chen, 2015).
Analyses and graphics were implemented in R 4.1.0 (R Core Team 2021). The structural equation model was implemented with the “lavaan” package, and all figures were created using the “ggplot2” and “ggpubr” packages.
3 Results
3.1 Characteristics of aboveground biomass of all woody individuals, multiple plant diversity, and stand structural attributes, and soil nutrients
Aboveground biomass of all woody individuals and big-sized trees, DBH variation, and soil nutrients displayed no significant variation across increasing scales (Figures 2A, B, I; Table S1). Neighborhood competition intensity of big-sized trees, species richness, and phylogenetic diversity exhibited increasing trends as spatial scales increased (Figures 2C, E, F; Table S1). Functional dispersion and CWM of leaf phosphorus concentration displayed decreasing trends with increasing scales (Figures 2G, H; Table S1).
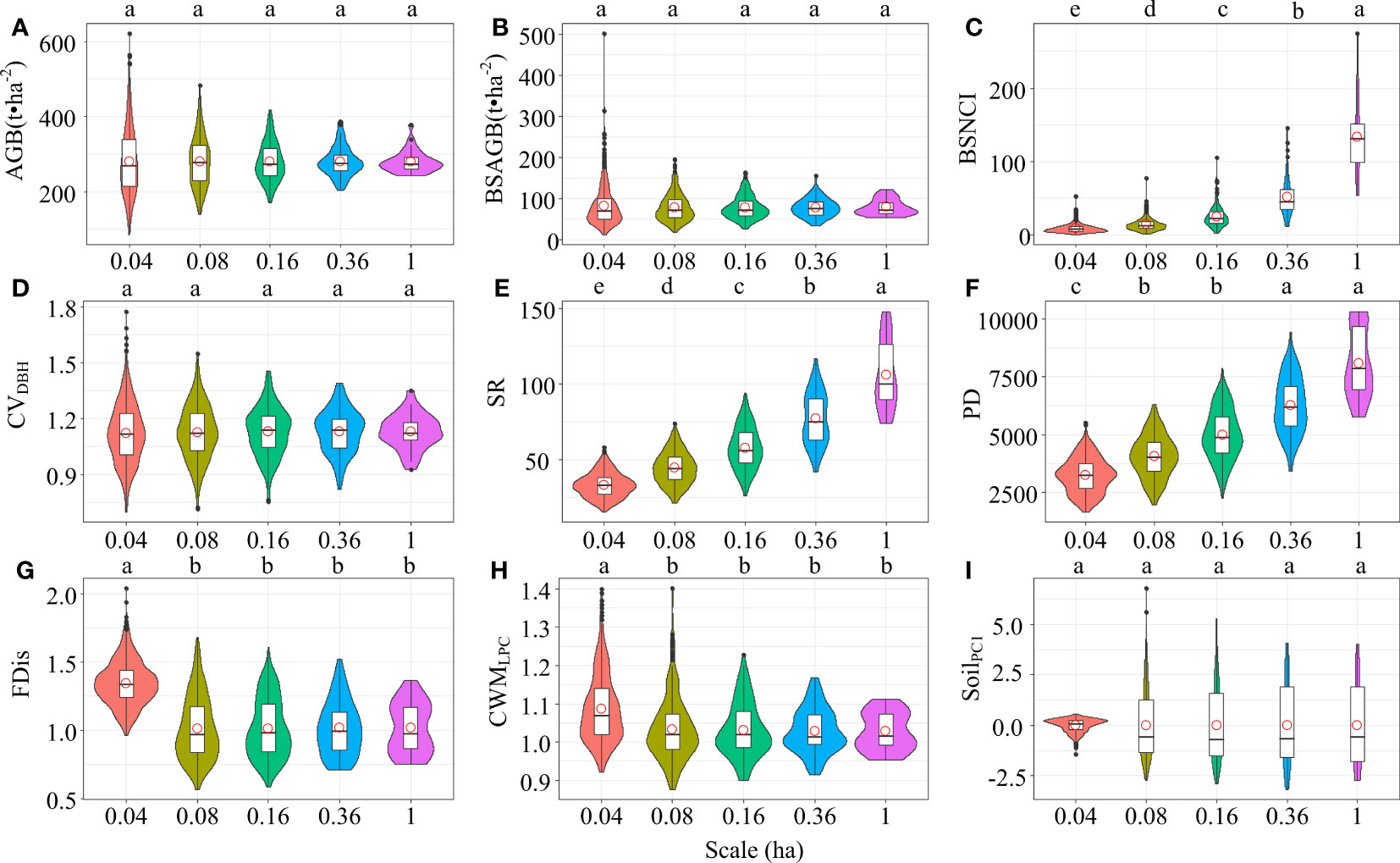
Figure 2 Comparison of (A) aboveground biomass of all woody individuals (AGB), (B) aboveground biomass of big-sized trees (BSAGB), (C) neighborhood competition intensity of big-sized trees (BSNCI), (D) DBH variation (CVDBH), (E) species richness (SR), (F) phylogenetic diversity (PD), (G) functional dispersion (FDis), (H) community-weighted mean of leaf phosphorus concentration (CWMLPC), and (I) soil nutrients (SoilPC1) based on violin plots across five spatial scales. Different letter in each violin plot exhibits difference significantly at P<0.05.
3.2 Bivariate relationships between aboveground biomass of all woody individuals and explanatory variables
Aboveground biomass of all woody individuals was influenced by effects of scale-dependent abiotic and biotic factors (Figures 3A–H), with aboveground biomass of all woody individuals demonstrating a positive linear trend with increasing species richness at the smallest scale (0.04ha), and no significant relationships with increasing scales (Figure 3A). Aboveground biomass of all woody individuals was not significantly related to either functional dispersion or phylogenetic diversity (Figures 3B, C). Aboveground biomass of all woody individuals decreased significantly with increasing CWM of leaf phosphorus concentration at smaller scales (Figure 3D, 0.04ha and 0.08ha), which decreased significantly with neighborhood competition intensity of big-sized trees at the scale of 0.04ha (Figure 3E). Aboveground biomass of all woody individuals increased significantly with aboveground biomass of big-sized trees (Figure 3F) and DBH variation (Figure 3G), which increased significantly with increasing soil nutrients at larger spatial scales (Figure 3H).
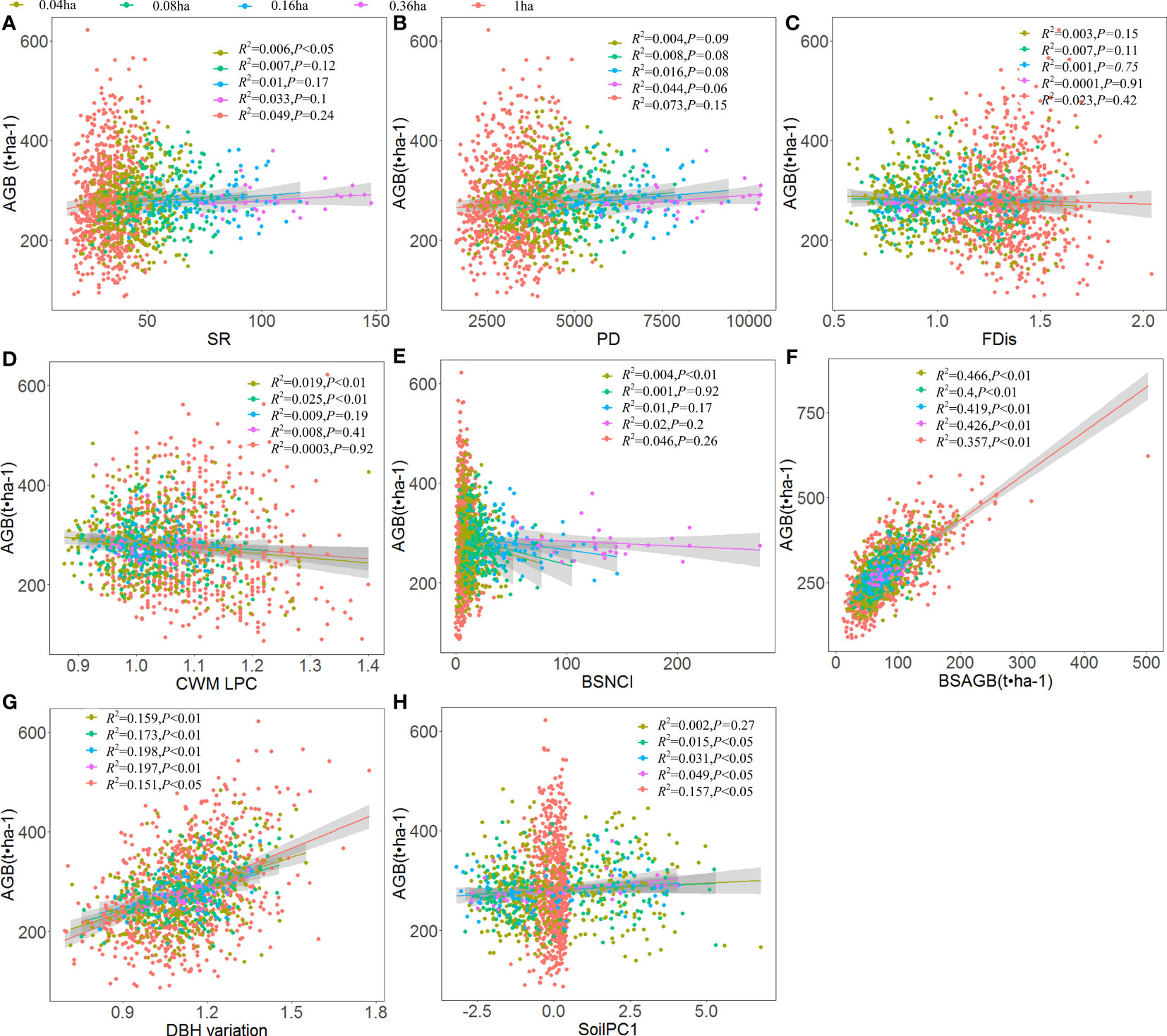
Figure 3 Relationships between aboveground biomass of all woody individuals (AGB) and (A) species richness (SR), (B) phylogenetic diversity (PD), (C) functional dispersion (FDis), (D) community-weighted mean of leaf phosphorus concentration (CWMLPC), (E) neighborhood competition intensity of big-sized trees (BSNCI), (F) aboveground biomass of big-sized trees (BSAGB), (G) DBH variation, and (H) soil nutrients (SoilPC1) using ordinary least linear regression at five scale-dependent changes including 0.04ha, 0.08ha, 0.16ha, 0.36ha, and 1ha.
3.3 Relative importance of plant diversity, big-sized trees, stand structural complexity, and soil nutrients on aboveground biomass of all woody individuals
Aboveground biomass of big-sized trees displayed the strongest effect on aboveground biomass of all woody individuals across all spatial scales (Figures 4A–E). DBH variation had the second greatest contribution at smaller scales from 0.04ha to 0.16ha, which then decreased at the scale of 0.36ha. Neighborhood competition intensity of big-sized trees contributed more to aboveground biomass of all woody individuals at the smallest scale, with minor contributions at larger scales. In addition, functional dispersion and CWM of leaf phosphorus concentration displayed more contributions to aboveground biomass of all woody individuals.
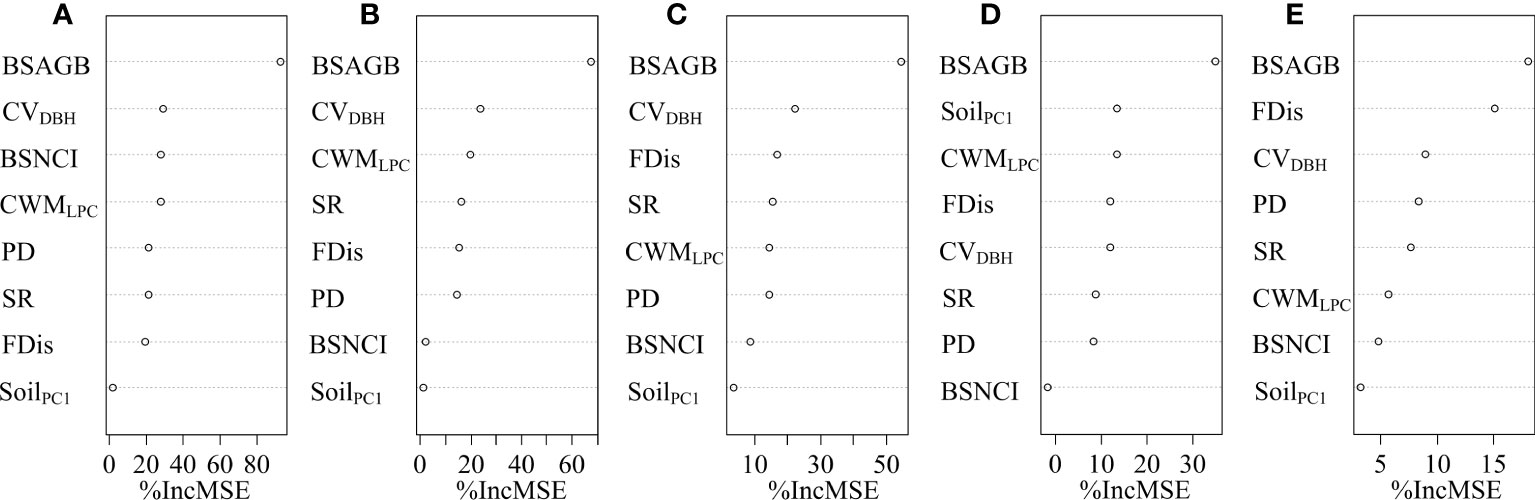
Figure 4 Main predictors of aboveground biomass of all woody individuals based on the random forest model at five sampling scales: (A) 0.04ha, (B) 0.08ha, (C) 0.16ha, (D) 0.36ha, and (E) 1ha. BSAGB, big-sized trees aboveground biomass; CVDBH, DBH variation; BSNCI, big-sized trees neighborhood competition intensity; SR, species richness; PD, phylogenetic diversity; FDis, functional dispersion; CWMLPC, community-weighted mean of leaf phosphorus concentration; SoilPC1, soil nutrients; %IncMSE, %Increase in mean squared error.
3.4 Effects of plant diversity, big-sized trees, stand structural complexity, and soil nutrients on aboveground biomass of all woody individuals
Based on the SEM showed that 55.7%, 50.8%, 54.7%, 59.6% and 72.9% of the variation in aboveground biomass of all woody individuals were explained by multiple plant diversity attributes, soil nutrients, DBH variation, neighborhood competition intensity and aboveground biomass of big-sized trees at five spatial scales, respectively (Figures 5A–E). CWM of leaf phosphorus concentration displayed a direct negative effect on aboveground biomass of all woody individuals across the five spatial scales, while species richness and phylogenetic diversity indicated no significant direct effects. Neighborhood competition intensity of big-sized trees had a stronger direct effect on aboveground biomass of all woody individuals at the smallest scale (0.04ha), and indicated indirect effects at the other scales via aboveground biomass of big-sized trees. Simultaneously, aboveground biomass of big-sized trees exhibited direct effects on aboveground biomass of all woody individuals across all spatial scales. In addition, DBH variations exhibited indirect effects on aboveground biomass of all woody individuals via neighborhood competition intensity and aboveground biomass of big-sized trees. Soil nutrients displayed no significant effects at most spatial scales.
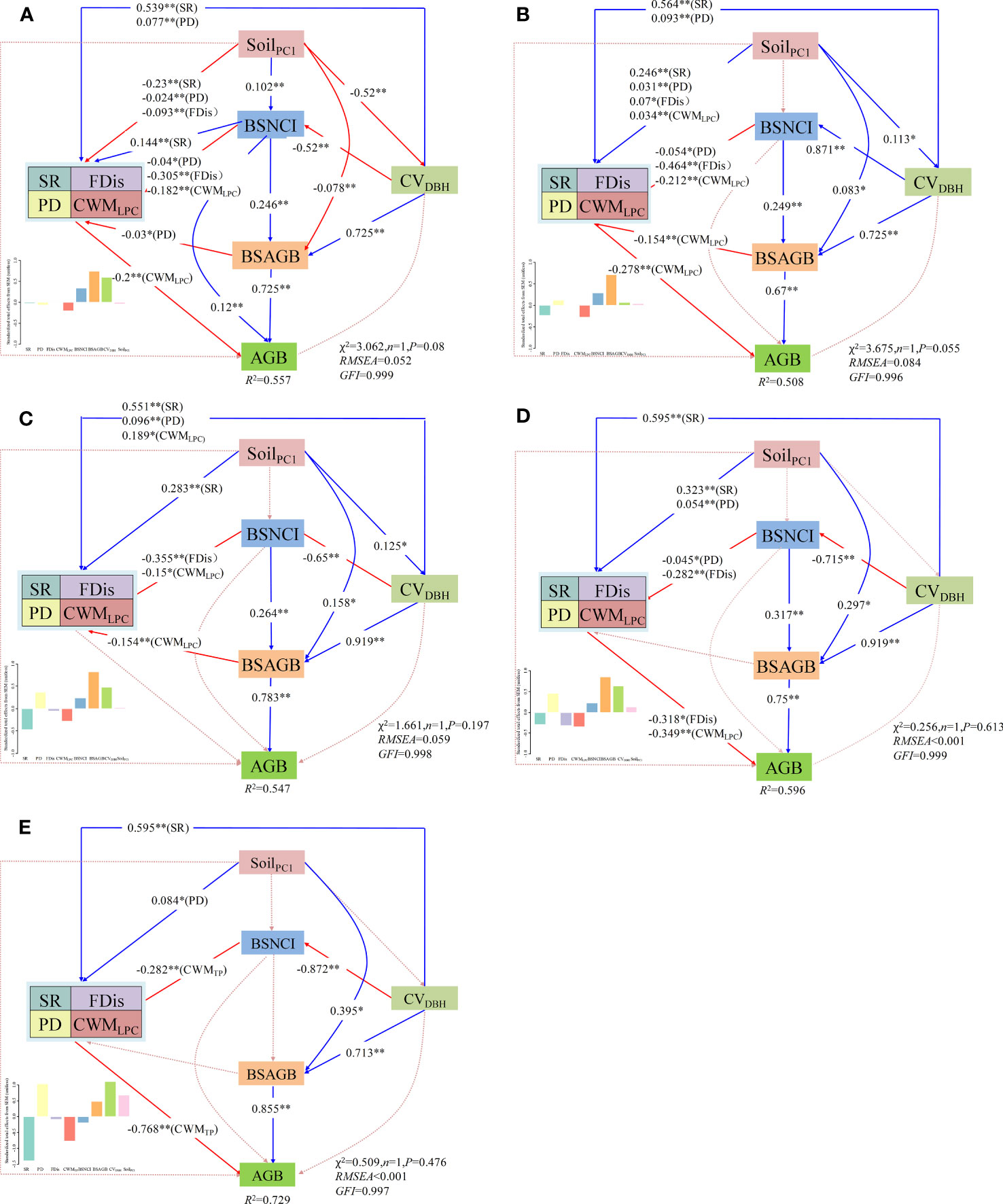
Figure 5 Structural equation models depicting direct and indirect effects of species richness (SR), functional dispersion (FDis), phylogenetic diversity (PD), community-weighted mean of leaf phosphorus concentration (CWMLPC), neighborhood competition intensity of big-sized trees (BSNCI), aboveground biomass of big-sized trees (BSAGB), DBH variation (CVDBH), and soil nutrients (soilPC1) on aboveground biomass of all woody individuals (AGB) at five sampling scales: (A) 0.04ha, (B) 0.08ha, (C) 0.16ha, (D) 0.36ha, and (E) 1ha. Blue solid arrows indicated significant positive effects, while red solid arrows indicated significant negative effects with standardized coefficients and significance (*P<0.05, **P<0.01). Red dashed arrows suggested no significant effects. Standardized total effects of abiotic and biotic factors on AGB from structural equation models are shown in the figure.
4 Discussion
In this study, we observed that plant species diversity exhibited a fewer contribution to aboveground biomass of all woody individuals across increasing spatial scales from 0.04 ha to 1 ha, while neighborhood competition intensity, aboveground biomass of big-sized trees and stand structural complexity displayed greater contribution. These results support that big-sized trees and stand structural complexity could affect the biodiversity–ecosystem functioning relationship (Zhang and Chen, 2015; Ali et al., 2019; Ali and Wang, 2021; Ali and Wang, 2021; Yuan et al., 2021). We found that aboveground biomass of all woody individuals increased with increasing plant species richness at the smallest spatial scale (0.04ha), while this relationship weakened or even disappeared with increasing spatial scales. Our study supported the first hypothesis that the positive relationship between species richness and aboveground biomass of all woody individuals decreased with increasing spatial scales. Previous studies found that the positive relationship between aboveground biomass and species richness is ubiquitous in forest ecosystems (Wu et al., 2015; Liang et al, 2016; Li SF et al., 2018; Liu et al., 2018). Our findings also confirmed that plant species richness was the main predictor in driving aboveground biomass at the plot scale threshold from 400 m2 (Yuan et al., 2019; Li et al, 2021), 600 m2 (Wu et al., 2015; Zhang and Chen, 2015), to 900 m2 (Liang et al., 2016; Liu et al., 2018). The scale-dependent result in our study was consistent with similar studies, which suggested that plant species richness had a positive effect on aboveground biomass at small spatial scale (400 m2) (Chisholm et al., 2013; Gonzalez et al., 2020). The species pool or heterogeneity varied independently of productivity, and a non-significant species richness–productivity relationship was found at larger spatial scales (Šímová et al., 2013). Our findings suggested that higher plant species richness was likely affected by habitat heterogeneity at larger spatial scales, while habitat heterogeneity did not depend on plant productivity.
The existence of scale dependencies in our findings suggest that the species richness–aboveground biomass relationship was greatly influenced by other biotic and abiotic factors (Fan et al., 2020; Gonzalez et al., 2020). This could be potentially explained by variation in ecosystem properties at different spatial scales. For example, plant species richness, phylogenetic diversity, and neighborhood competition intensity of big-sized trees increased with increasing spatial scale, while bias of aboveground biomass of all woody individuals became more stability with increasing spatial scale (Figure 2). In fact, the positive relationship between plant species richness and aboveground biomass of all woody individuals at small spatial scales may be mediated by stem density rather than selection and complementary effects (Chisholm et al., 2013). Moreover, plant species richness exhibited no significant direct effect on aboveground biomass of all woody individuals based on the structural equation modeling across all spatial scales when other response variables were considered.
Across all five spatial scales, aboveground biomass of big-sized trees exhibited a consistently positive effect on aboveground biomass of all woody individuals, suggesting that big-sized trees are a strong driver in accumulating aboveground biomass of all woody individuals in a tropical montane evergreen broad-leaved forest. It is not surprising that aboveground biomass of big-sized trees strongly affected aboveground biomass of all woody individuals according to a simple power law relationship with tree size. Big-sized trees displayed a disproportionate contribution to stand-level productivity (Yuan et al., 2021), and fewer big-sized trees contributed a larger fraction to aboveground biomass of all woody individuals (Lohbeck et al., 2016; Ali and Wang, 2021). These results emphasize the importance of the “big-sized trees hypothesis” in sustaining ecosystem function, even though small- and medium-sized trees contribute substantially to plant diversity and soil carbon cycling in tropical forests (Ali et al., 2019). In a tropical montane evergreen broad-leaved forest, big-sized trees included slow-growing species, such as Castanopsis echinocarpa, Lithocarpus truncates, Lithocarpus fenestratus, and Schima wallichii, as well as fast-growing species, such as Alnus nepalensis, Lindera metcalfiana var. dictyophylla, and Betula alnoides. The larger DBH of these species demonstrated greater resource availability for biomass accumulation, including light-use efficiency and soil nutrients uptake (Lohbeck et al., 2016; Ali and Wang, 2021; Yuan et al., 2021), which have become stronger predictors of aboveground biomass of all woody individuals in the stand level, which have played a vital role in sustaining ecosystem function.
Our findings suggest that selection and complementarity effects also played an important role in accumulating aboveground biomass of all woody individuals. Stand structural complexity displayed positive effect on aboveground biomass of all woody individuals indirectly through aboveground biomass of big-sized trees across all spatial scales, which suggested that complementarity effects could maintain higher plant species richness resulting in niche differentiation and facilitation (Zhang and Chen, 2015; Ali et al., 2019; Mensah et al., 2020; Yuan et al., 2021). Big-sized trees further supported the selection effect that larger productive and functioning species can result in higher ecosystem provisioning (Loreau and Hector, 2001; Šímová et al., 2013), which could serve as a selection mechanism for more competitive species from an abundant species pool with higher competition intensity. Moreover, complementarity effect increased with competition intensity, which could improve individual tree productivity (Searle and Chen, 2020). In our study, big-sized trees indicated complementary light use strategies among neighboring trees (Jucker et al., 2014), which exhibited weaker competition with small- and medium-sized woody species for light and water by niche differentiation. Competition intensity played a crucial factor in driving and regulating aboveground biomass allocation (Jucker et al., 2014; Zhou et al., 2018; Mahaut et al., 2020). Neighborhood competition intensity of big-sized trees displayed a positive effect on aboveground biomass of all woody individuals at the smallest spatial scale, as well as an indirect positive influence on aboveground biomass of all woody individuals through aboveground biomass of big-sized trees. These finding supports our third hypothesis that neighborhood competition intensity of big-sized trees regulated the relationship between plant diversity and aboveground biomass of all woody individuals.
Our finding showed that CWM of leaf phosphorus concentration demonstrated a negative effect on aboveground biomass of all woody individuals at most spatial scales, which was inconsistent with previous studies (Finegan et al., 2015; Ali et al., 2017). In forest ecosystems, with high plant species richness and complex community structure, it can be difficult to determine which composition of traits could predict aboveground biomass of all woody individuals with high plant species richness and complex community structure. Functional traits are a promising tool for exploring diversity–biomass relationship (Mahaut et al., 2020), and functional trait compositions could enhance uptake of nutrients, such as phosphorus, by trait complementarity in light, water, and nutrient interception (De Deyn et al., 2008). Higher productivity maybe depend on resource conservative trees (Yuan et al., 2021), such as trees with lower leaf phosphorus concentrations. There are a large number of small- and medium-sized woody species in this tropical montane evergreen broad-leaved forest (Li et al., 2020b), and growth of these species has been shown to be mainly influenced by soil phosphorus (Li SF et al., 2018). Regardless, big-sized trees were the main contributor to aboveground biomass of all woody individuals, which may serve as one explanation for the lower CWM of leaf phosphorus concentration with higher aboveground biomass. This could be because dominant species traits may determine ecosystem function based on the mass-ratio hypothesis (Finegan et al., 2015; Lohbeck et al., 2016; Yuan et al., 2021). Our results strongly suggest that CWM of leaf phosphorus concentration of larger woody individuals is a more important predictor of aboveground biomass than CWM of leaf phosphorus concentration of all woody individuals in the tropical montane evergreen broad-leaved forest.
Although previous studies suggested that soil nutrients play a vital role in driving aboveground biomass (van der Sande et al., 2018; Yuan et al., 2019), our study did not support given the scale-dependent results. One possible explanation could be that the magnitude and stability of ecosystem functioning depends strongly on the degree of autocorrelation in the environment (Gonzalez et al., 2020). Slower rate of species turnover on account of similar soil nutrients may be attributable to a negligible effect on accumulating aboveground biomass of all woody individuals in the 30ha dynamics plot. However, soil nutrients indirectly affected aboveground biomass accumulation of all woody individuals through big-sized trees, which suggested that big-sized trees with deeper roots are more susceptible to minor soil nutrient variability and improve aboveground biomass accumulation with greater soil nutrients availability (Yuan et al., 2019; Ali and Wang, 2021).
The present study provided evidence to support that big-sized trees attributes and functional diversity were more important roles than other factors including soil nutrients and multiple biodiversity attributes in determining accumulating aboveground biomass in a tropical montane evergreen broad-leaved forest. Moreover, both selection effects and complementarity effects could explain the variation in accumulating aboveground biomass of all woody individuals, and complementarity effects played a more important role in accumulating aboveground biomass of big-sized trees than selection effects. These results suggested that the two underlying mechanisms are not contradictory, and that they may play an irreplaceable role in accumulating aboveground biomass in a tropical montane evergreen broad-leaved forest. Our study strengthens the importance of neighborhood competition intensity in accumulating aboveground biomass, and stand structural complexity indicated an indirect effect on aboveground biomass. Our findings provide meaningful suggestions for afforestation and forest management that big-sized trees should be retained and protected in considering the biodiversity–ecosystem function relationship.
Data availability statement
The raw data supporting the conclusions of this article will be made available by the authors, without undue reservation.
Author contributions
SL and JS conceived the study and designed the methodology. SL, XL, XH, YW, RT, WL, and CX collected the data. SL analyzed the data and wrote the manuscript. All authors contributed critically to the drafts and gave final approval for publication.
Funding
This study was funded by the Fundamental Research Funds of CAF (CAFYBB2020ZA002 and CAFYBB2021ZA002).
Acknowledgments
We thank strong assistance of Taiyanghe Provincial Nature Reserve bureau. We could like to thank Dr. Shannon Elliot at Michigan State University for his assistance with English language and grammatical editing.
Conflict of interest
The authors declare that the research was conducted in the absence of any commercial or financial relationships that could be construed as a potential conflict of interest.
Publisher’s note
All claims expressed in this article are solely those of the authors and do not necessarily represent those of their affiliated organizations, or those of the publisher, the editors and the reviewers. Any product that may be evaluated in this article, or claim that may be made by its manufacturer, is not guaranteed or endorsed by the publisher.
Supplementary material
The Supplementary Material for this article can be found online at: https://www.frontiersin.org/articles/10.3389/fevo.2023.1188161/full#supplementary-material
References
Ali A. (2019). Forest stand structure and functioning: current knowledge and future challenges. Ecol. Indic. 98, 665–677. doi: 10.1016/j.ecolind.2018.11.017
Ali A., Lin S. L., He J. K., Kong F. M., Yu J. H., Jiang H. S. (2019). Big-sized trees overrule remaining tree’s attributes and species richness as determinants of aboveground biomass in tropical forests. Global Change Biol. 25, 2810–2824. doi: 10.1111/gcb.14707
Ali A., Wang L. Q. (2021). Big-sized trees and forest functioning: current knowledge and future perspectives. Ecol. Indic. 127, 107760. doi: 10.1016/j.ecolind.2021.107760
Ali A., Yan E. R., Chang S. X., Chen J. Y., Liu X. Y. (2017). Community-weighted mean of leaf traits and divergence of wood traits predict aboveground biomass in secondary subtropical forests. Sci. Total Environ. 574, 654–662. doi: 10.1016/j.scitotenv.2016.09.022
Barry K. E., Pinter G. A., Strini J. W., Yang K., Lauko I. G., Schnitzer S. A., et al. (2021). A graphical null model for scaling biodiversity-ecosystem functioning relationships. J. Ecol. 109, 1549–1560. doi: 10.1111/1365-2745.13578
Cardinale B. J., Palmer M. A., Collins S. L. (2002). Species diversity enhances ecosystem functioning through interspecific facilitation. Nature 415, 426–429. doi: 10.1038/415426a
Chalcraft D. R., Williams J. W., Smith M. D., Willig M. R. (2004). Scale dependence in the species-richness-productivity relationship: the role of species turnover. Ecology 85, 2701–2708. doi: 10.1890/03-0561
Chave J., Réjou-Méchain M., Búrquez A., Chidumayo E., Colgan M. S., Delitti W. B. B., et al. (2014). Improved allometric models to estimate the aboveground biomass of tropical trees. Global Change Biology 20, 3177–3190. doi: 10.1111/gcb.12629
Chisholm R. A., Muller-Landau H. C., Rahman K. A., Bebber D. P., Bin Y., Bohlman S. A., et al. (2013). Scale-dependent relationships between tree species richness and ecosystem functions in forests. J. Ecol. 101, 1214–1224. doi: 10.1111/1365-2745.12132
Clark J. S. (2010). Individuals and the variation needed for high species diversity in forest tree. Science 327, 1129–1132. doi: 10.1126/science.1183506
Craven D., van der Sande M. T., Meyer C., Gerstner K., Bennett J. M., Giling D. P., et al. (2020). A cross-scale assessment of productivity-diversity relationships. Global Ecol. Biogeography 29, 1940–1195. doi: 10.1111/geb.13165
De Andrés E. G., Camarero J. J., Blanco J. A., Imbert J. B., Lo Y. H., Sangüesa-Barreda G., et al. (2018). Tree-to-tree competition in mixed European beech-Scots pine forests has different impacts on growth and water-use efficiency depending on site conditions. J. Ecol. 106, 59–75. doi: 10.1111/1365-2745.12813
De Deyn G. B., Cornelissen H. C., Bardgett R. D. (2008). Plant functional tratis and soil carbon sequestration in contrasting biomes. Ecol. Lett. 11, 516–531. doi: 10.1111/j.1461-0248.2008.01164.x
Fan C. Y., Tan L. Z., Zhang C. Y., Zhao X. H., Gao L. S., von Gadow K. (2020). Scale-dependent effects of neighborhood biodiversity on individual tree productivity in a coniferous and broad-leaved mixed forest in China. Ecol. Evol. 10, 8225–8234. doi: 10.1002/ece3.6530
Finegan B., Peña-Claros M., de Oliveria A., Ascarrunz N., Bret-Harte M. S., Carreño-Rocabado G., et al. (2015). Does functional trait diversity predict above-ground biomass and productivity of tropical forests? Testing tree alternative hypotheses. J. Ecol. 103, 191–201. doi: 10.1111/1365-2745.12346
Flynn D. F. B., Mirotchnick N., Jain M., Palmer M. I., Naeem S. (2011). Functional and phylogenetic diversity as predictors of biodiversity-ecosystem-function relationships. Ecology 92, 1573–1481. doi: 10.1890/10-1245.1
Gonzalez A., Germain R. M., Srivastava D. S., Filotas E., Dee L. E., Gravel D., et al. (2020). Scaling-up biodiversity-ecosystem functioning research. Ecol. Lett. 23, 757–779. doi: 10.1111/ele.13456
González-Caro S., Duivenvoorden J. F., Balslev H., Cavelier J., Grández C., Macía M. J., et al. (2021). Scale-dependent drivers of the phylogenetic structure and similarity of tree communities in northwestern Amazonia. J. Ecol. 109, 888–899. doi: 10.1111/1365-2745.13514
Grace J. B., Anderson T. M., Seabloom E. W., Borer E. T., Adler P. B., Harpole W. S., et al. (2016). Integrative modelling reveals mechanisms linking productivity and plant species richness. Nature 529, 390–393. doi: 10.1038/nature16524
Hegyi F. (1974). “A simulation model for managing jack pine stands,” in Growth models for tree and stand simulations. Ed. Fried J. (Stokholm: Royal College of Forestry), 74–90.
Hui G. Y., Wang Y., Zhang G. Q., Zhao Z. H., Bai C., Liu W. Z. (2018). A novel approach for assessing the neighborhood competition in two different aged forests. For. Ecol. Manage. 422, 49–58. doi: 10.1016/j.foreco.2018.03.045
Jing X., Muys B., Bruelheide H., Desie E., Hättenschwiler S., Jactel H., et al. (2021). Above- and below-ground complementarity rather than selection drive tree diversity-productivity relationships in European forests. Funct. Ecol. 35, 1756–1767. doi: 10.1111/1365-2435.13825
Jucker T., Bouriaud O., Avacaritei D., Dănilă L., Duduman G., Valladares F., et al. (2014). Competition for light and water play contrasting roles in driving diversity-productivity relationships in Iberian forests. J. Ecol. 102, 1202–1213. doi: 10.1111/1365-2745.12276
Kunz M., Fichtner A., Härdtle W., Raumonen P., Bruelheide H., von Oheimb G. (2019). Neighbour species richness and local structural variability modulate aboveground allocation patterns and crown morphology of individual trees. Ecol. Lett. 22, 2130–2140. doi: 10.1111/ele.13400
Li Y., Bao W. K., Bongers F., Chen B., Chen G. K., Guo K., et al. (2019). Drivers of tree carbon storage in subtropical forests. Sci. Total Environ. 654, 684–693. doi: 10.1016/j.scitotenv.2018.11.024
Li S. F., Huang X. B., Lang X. D., Shen J. Y., Xu F. D., Su J. R. (2020a). Cumulative effects of multiple biodiversity attributes and abiotic factors on ecosystem multifunctionality in the Jinsha River valley of southwestern China. For. Ecol. Manage. 472, 118281. doi: 10.1016/j.foreco.2020.118281
Li S. F., Lang X. D., Huang X. B., Wang Y. H., Liu W. D., Xu C. H., et al. (2020b). Association classification of a 30hm2 dynamics plots in the monsoon broad-leaved evergreen forest in Pu’er, Yunnan, China. Chinese. J. Plant Ecol. 44, 236–247. doi: 10.17521/cjpe.2019.0268
Li S. F., Lang X. D., Liu W. D., Ou G. L., Xu H., Su J. R. (2018). The relationship between species richness and aboveground biomass in a primary Pinus kesiya forest of Yunnan, southwestern China. PloS One 13, e0191140. doi: 10.1371/journal.pone.0191140
Li S. F., Liu W. D., Lang X. D., Huang X. B., Su J. R. (2021). Species richness, not abundance, drives ecosystem multifunctionality in a subtropical coniferous forest. Ecol. Indic. 120, 106911. doi: 10.1016/j.ecolind.2020.106911
Li Y., Tian D. S., Yang H., Niu S. L. (2018). Size-dependent nutrient limitation of tree growth from subtropical to cold temperate forests. Funct. Ecol. 32, 95–105. doi: 10.1111/1365-2435.12975
Liang J. J., Crowther T. W., Picard N., Wiser S. N., Zhou M., Alberti G., et al. (2016). Positive biodiversity-productivity relationship predominant in global forests. Science 354, aaf8957. doi: 10.1126/science.aaf8957
Liang M. X., Liu X. B., Parker I. M., Johnson D., Zheng Y., Luo S., et al. (2019). Soil microbes drive phylogenetic diversity-productivity relationships in a subtropical forest. Sci. Adv. 5, eaax5088. doi: 10.1126/sciadv.aax5088
Liu X. J., Trogisch S., He J. S., Niklaus P. A., Bruelheide H., Tang Z. Y., et al. (2018). Tree species richness increases ecosystem carbon storage in subtropical forests. Proc. R. Soc. B: Biol. Sci. 285, 20181240. doi: 10.1098/rspb.2018.1240
Lohbeck M., Bongers F., Martinez-Ramos M., Poorter L. (2016). The importance of biodiversity and dominance for multiple ecosystem functions in a human-modified tropical landscape. Ecology 97, 2772–2779. doi: 10.1002/ecy.1499
Loreau M., Hector A. (2001). Partitioning selection and complementarity in biodiversity experiments. Nature 412, 72–76. doi: 10.1038/35083573
Luo Y. H., Cadotte M. W., Burgess K. S., Liu J., Tan S. L., Zou J. Y., et al. (2019). Greater than the sum of the parts: how the species composition in different forest strata influence ecosystem function. Ecol. Lett. 22, 1449–1461. doi: 10.1111/ele.13330
Mahaut L., Fort F., Violle C., Freschet G. T. (2020). Multiple facets of diversity effects on plant productivity: species richness, functional diversity, species identity and intraspecific competition. Funct. Ecol. 34, 287–298. doi: 10.1111/1365-2435.13473
McDowell N. G., Allen C. D., Anderson-Teixeira K., Aukema B. H., Bond-Lamberty B., Chini L., et al. (2020). Pervasive shifts in forest dynamics in a changing world. Science 368, eaaz9463. doi: 10.1126/science.aaz9463
Mensah S., Salako V. K., Seifert T. (2020). Structural complexity and large-sized trees explain shifting species richness and carbon relationship across vegetation types. Funct. Ecol. 34, 1731–1745. doi: 10.1111/1365-2435.13585
Ni J., Wang G. H., Bai Y. F., Li X. Z. (2007). Scale-dependent relationships between plant diversity and above-ground biomass in temperature grasslands, south-eastern Mongolia. J. Arid Environments 68, 132–142. doi: 10.1016/j.jaridenv.2006.05.003
Ordoñez J. C., van Bodegom P. M., Witte J. P. M., Wright I. J., Reich P. B., Aerts R. (2009). A global study of relationships between leaf traits, climate and soil measures of nutrient fertility. Global Ecol. Biogeography 18, 137–149. doi: 10.1111/j.1466-8238.2008.00441.x
R Core Team. (2021). R: A Language and Environment for Statistical Computing. (Vienna: R Foundation for Statistical Computing).
Rosseel Y. (2012). Lavaan: an R package for structural equation modeling. J. Stat. Software 48, 1–36. doi: 10.18637/jss.v048.i02
Schnitzer S. A., Klironomos J. N., HilleRisLambers J., Kinkel L. L., Reich P. B., Xiao K., et al. (2011). Soil microbes drive the classic plant diversity-productivity pattern. Ecology 92, 296–303. doi: 10.1890/10-0773.1
Searle E. B., Chen H. Y. H. (2020). Complementarity effects are strengthened by competition intensity and global environmental change in the central boreal forests of Canada. Ecol. Lett. 23, 79–87. doi: 10.1111/ele.13411
Šímová I., Li Y. M., Storch D. (2013). Relationship between species richness and productivity in plants: the role of sampling effect, heterogenetiy and species pool. J. Ecol. 101, 161–170. doi: 10.1111/1365-2745.12011
Steur G., Verburg R. W., Wassen M. J., Verweij P. A. (2020). Shedding light on relationships between plant diversity and tropical forest ecosystem services across spatial scales and plot sizes. Ecosystem Serv. 43, 101107. doi: 10.1016/j.ecoser.2020.101107
van der Heijden M. G. A., Bardgett R. D., van Straalen N. M. (2008). The unseen majority: soil microbes as drivers of plant diversity and productivity in terrestrial ecosystems. Ecol. Lett. 11, 296–310. doi: 10.1111/j.1461-0248.2007.01139.x
van der Sande M. T., Arets E. J. M. M., Peña-Claros M., Hoosbeek M. R., Cáceres-Siani Y., van der Hout P., et al. (2018). Soil fertility and species traits, but not diversity, drive productivity and biomass stocks in a Guyanese tropical rainforest. Funct. Ecol. 32, 461–474. doi: 10.1111/1365-2435.12968
van der Sande M. T., Pena-Claros M., Ascarrunz N., Arets E. J. M. M., Licona J. C., Toledo M., et al. (2017). Abiotc and biotic drivers of biomass change in a Neotropical forest. J. Ecol. 105, 1223–1234. doi: 10.1111/1365-2745.12756
Villa P. M., Ali A., Martins S. V., de Oliveira Neto S. N., Rodrigues A. C., Teshome M., et al. (2020). Stand structural attributes and functional trait compostion overrule the effects of functional divergence on aboveground biomass during Amazon forest succession. For. Ecol. Manage. 477, 118481. doi: 10.1016/j.foreco.2020.118481
Wu X., Wang X. P., Tang Z. Y., Shen Z. H., Zheng C. Y., Xia X. L., et al. (2015). The relationship between species richness and biomass changes from boreal to subtropical forests in China. Ecography 38, 602–613. doi: 10.1111/ecog.00940
Yuan Z. Q., Ali A., Jucker T., Ruiz-Benito P., Wang S., Jiang L., et al. (2019). Multiple abiotic and biotic pathways shape biomass demographic processes in temperate forests. Ecology 100, e02650. doi: 10.1002/ecy.2650
Yuan Z. Q., Ali A., Sanaei A., Ruiz-Benito P., Jucker T., Fang L., et al. (2021). Few large trees, rather than plant diversity and composition, drive the above-ground biomass stock and dynamics of temperate forests in northeast China. For. Ecol. Manage. 481, 118698. doi: 10.1016/j.foreco.2020.118698
Zhang Y., Chen Y. H. (2015). Individual size inequality links forest diversity and above-ground biomass. J. Ecol. 103, 1245–1252. doi: 10.1111/1365-2745.12425
Zhou W. S., Cheng X. Q., Wu R., Han H. R., Kang F. F., Zhu J., et al. (2018). Effect of intraspecific competition on biomass partitioning of Larix principis-rupprechtii. J. Plant Interact. 13, 1–8. doi: 10.1080/17429145.2017.1406999
Keywords: species richness, functional dispersion, phylogenetic diversity, soil nutrients, neighborhood competition intensity, biodiversity and ecosystem functioning relationship, scale dependence
Citation: Li S, Lang X, Huang X, Wang Y, Tang R, Liu W, Xu C and Su J (2023) Effects of plant diversity and big-sized trees on ecosystem function in a tropical montane evergreen broad-leaved forest. Front. Ecol. Evol. 11:1188161. doi: 10.3389/fevo.2023.1188161
Received: 17 March 2023; Accepted: 01 August 2023;
Published: 16 August 2023.
Edited by:
Zuoqiang Yuan, Northernwest Polytechnical University, ChinaReviewed by:
Jacqueline R. England, Commonwealth Scientific and Industrial Research Organisation (CSIRO), AustraliaChang-Bae Lee, Kookmin University, Republic of Korea
Copyright © 2023 Li, Lang, Huang, Wang, Tang, Liu, Xu and Su. This is an open-access article distributed under the terms of the Creative Commons Attribution License (CC BY). The use, distribution or reproduction in other forums is permitted, provided the original author(s) and the copyright owner(s) are credited and that the original publication in this journal is cited, in accordance with accepted academic practice. No use, distribution or reproduction is permitted which does not comply with these terms.
*Correspondence: Jianrong Su, jianrongsu@vip.sina.com