Present and future distribution of Faidherbia albida in Cabo Verde as revealed by climatic modelling and LULC analysis
- 1Linking Landscape, Environment, Agriculture and Food (LEAF), Associated Laboratory TERRA, Instituto Superior de Agronomia (ISA), Universidade de Lisboa, Lisbon, Portugal
- 2Research Centre in Biodiversity and Genetic Resources – Azores (CIBIO-Açores), InBIO Associate Laboratory, Faculdade de Ciências e Tecnologia, Universidade dos Açores, Vairão, Portugal
- 3Nova School of Business and Economics, Carcavelos, Portugal
- 4Centro de Estudos Africanos Para Desenvolvimento e Inovação (CEADI), Assomada, Cabo Verde
- 5Centre for Ecology, Evolution and Environmental Changes (cE3c) and CHANGE - Global Change and Sustainability Institute, Faculdade de Ciências, Universidade de Lisboa, Lisbon, Portugal
- 6BIOPOLIS Program in Genomics, Biodiversity and Land Planning, CIBIO, Vairão, Portugal
- 7UNESCO Chair – Land Within Sea: Biodiversity and Sustainability in Atlantic Islands, Ponta Delgada, Azores, Portugal
Climate change poses one of the most significant challenges to conserve biodiversity, especially in tropical dry islands, as is the case of Cabo Verde (northeast Atlantic Ocean). This archipelago has a low percentage of forest cover and hosts only seven native tree species, among them, Faidherbia albida (Delile) A.Chev. (Fabaceae). Therefore, protective afforestation is extremely important in Cabo Verde, one of the most vulnerable West African countries to climate change. With this work, we aimed to estimate the current distribution and potential shifts in suitable areas for F. albida under climate change, using species distribution models (i.e., random forest, generalized linear and additive models), covering its distribution range in Cabo Verde and mainland Africa. The best model was then projected for the studied area, at two different slice times, using Representative Concentration Pathway (RCP) 4.5 and 8.5 scenarios. Based on current bioclimatic variables, we estimated that almost two thirds of Cabo Verde’s territory is highly suitable for F. albida, which contrasts with its current occurrence. By overlaying the present habitat suitability with land use and land cover data, we concluded that habitat availability and suitability could be constrained by that factor. On average, the predicted suitable habitat for future distributions gradually decreases by 2080 under both scenarios compared with the current, with a smaller effect of RCP4.5 than of RCP8.5. Local authorities can benefit from this research and develop actions to promote sustainable reforestation in Cabo Verde, which should include native tree species that are best adapted to the local climate and could thus contribute to mitigate the effects of climate change.
1. Introduction
There is a very high probability that global warming will reach or surpass 1.5°C in the near future (2030–2050), as a result of insufficient progress toward the Sustainable Development Goals (IPCC, 2022). The West African region is already experiencing a continuous warming trend, with a temperature increase of 0.2–0.5°C per decade in countries such as Ghana, Côte d’Ivoire, Guinea, and Senegal (Sylla et al., 2016). The vulnerability of these low-income African countries to natural disasters (e.g., drought) is particularly worrying in the context of climate change, mainly due to the importance of rainfed agriculture in the regional economy, and the low level of water management (Varela et al., 2020). Also, climate and land-use changes have significant impacts on the distribution of plant species (e.g., Parmesan and Hanley, 2015; Feeley et al., 2020).
Among the many semi-arid climates of Africa, most countries – including Cabo Verde islands, the focus of this study – have been heavily affected by drought over the last decades, with highly variable and low rainfall (Monteiro et al., 2020). This situation worsens in the climate change projections and will directly and indirectly affect species distribution and abundance at a global scale, as well as the growth and productivity of forests through changes in temperature, precipitation, and other climate-related factors (Chang et al., 2015; Ferrarini et al., 2019; Santini et al., 2021).
Located in the northeast Atlantic Ocean, Cabo Verde is located c. 500 km west of Senegal (West Africa) and is the southernmost archipelago encompassed by Macaronesia (i.e., Azores, Madeira, Savage Islands, Canary Islands, and Cabo Verde; Florencio et al., 2021). The wide variety of habitats within the Macaronesian Islands is home to a rich biodiversity, but this huge diversity is not properly understood, and many lineages remain understudied, in Cabo Verde (Romeiras et al., 2019). Particularly, the dry tropical forests and tree species of these islands are poorly known in comparison to those of the other Macaronesian archipelagos (Norder et al., 2020; Castilla-Beltran et al., 2021). There are only seven tree taxa native to Cabo Verde (Romeiras et al., 2016): three are endemic – Dracaena draco subsp. caboverdeana Marrero Rodr. & R.S.Almeida; Phoenix atlantica A.Chev; Sideroxylon marginatum (Decne. ex Webb) Cout.; and four are non-endemic – Faidherbia albida (Delile) A.Chev. (Family: Fabaceae Lindl.), Ficus sur Forssk., Ficus sycomorus L., and Tamarix senegalensis DC.
Effective protection, management, and future expansion of the remnants of Cabo Verde’s native forests with their full array of species depend on the basic knowledge of the factors constraining species distribution, as well as a comprehensive account of their current distribution and environmental suitability maps. Nowadays, Species Distribution Models (SDMs) emerged as useful tools in spatial ecology, conservation, and land management (e.g., Thuiller et al., 2011, 2016; Casajus et al., 2016) by establishing a connection between species occurrences (i.e., species presence or abundance) and a set of explanatory variables that are mostly environmental descriptors (e.g., bioclimatic variables, altitude, solar radiation, land cover/use, type of soil/substrate) that can then be projected under various climate scenarios (Silva et al., 2017, 2019, 2021). Moreover, SDMs generate geographical maps of environmental suitability that can be used to prioritize conservation areas, forecast the effects of climate change on species ranges, and assess the likelihood of biological invasions (e.g., Araújo et al., 2005; Casajus et al., 2016; Thuiller et al., 2020). There are numerous modelling techniques, including Random Forest (RF), Generalized Linear Models (GLM), and Generalized Additive Models (GAM; e.g., Breiman, 2001; Prasad et al., 2006; Wooldridge, 2008; Dobson and Barnett, 2018; Silva et al., 2021). These methods are reliable to model tree species distributions in Macaronesian islands, namely in Canary Islands (Arévalo et al., 2005) and Azores (Silva et al., 2017, 2019); recently, Varela et al. (2022) delimited the climatic niche of Cabo Verde endemics (i.e., Dracaena draco subsp. caboverdeana; Phoenix atlantica; Sideroxylon marginatum) and the effects of predicted climate change on their future distribution range.
To our knowledge, the factors determining the present and future distribution of the native non-endemic Cabo Verde trees, all with their native range in Africa, were not yet documented. Among them, the leguminous Faidherbia albida that is an important component of Cabo Verde’s native vegetation, and can be used in reforestation programs, as it is adapted to semiarid African habitats (Figure 1); it can thus contribute to biodiversity conservation in these islands, counteracting forest cover loss and promoting the cultivation of species resilient to climate change (Romeiras et al., 2016).
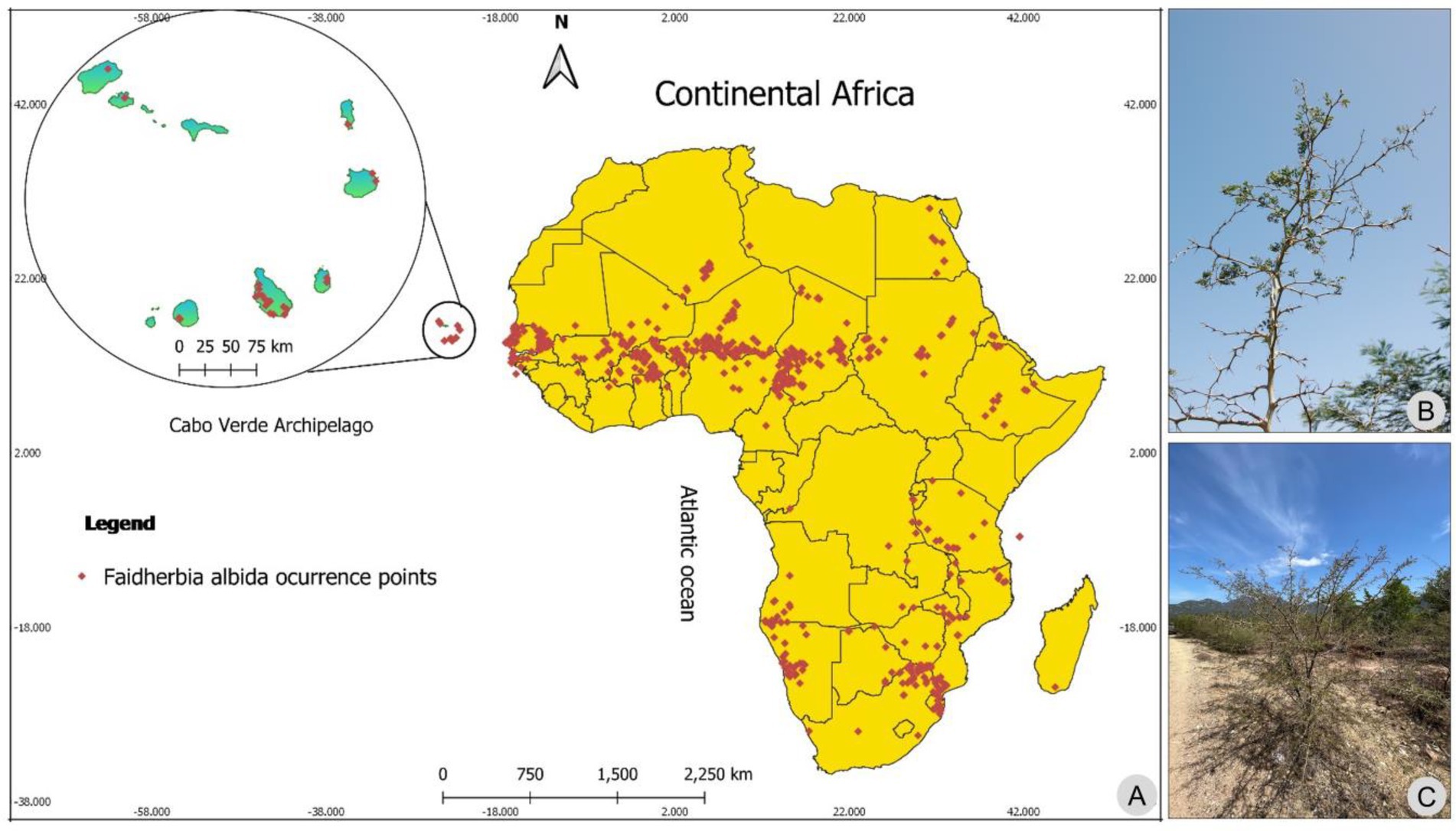
Figure 1. Occurrence points of Faidherbia albida in Africa mainland and Cabo Verde islands (A). Details of Faidherbia albida (B) and African woodlands (C). Photos by M.M. Romeiras.
Based on current and future climate scenarios, some African legume trees were recently studied (e.g., Adjonou et al., 2020; Atanasso et al., 2021; Catarino et al., 2021). For instance, Nyairo and Machimura (2020) revealed the range projections for nine taxa in Kenya, highlighting that a mix of expansion or contraction occurs, according to the species’ ability to establish in urban areas or inability to endure the predicted climate change. Furthermore, testing hypotheses on diversification processes, Gorel et al. (2019) suggested that ecological speciation through climate probably played a key role in the evolution of the tropical Leguminosae Erythrophleum genus, widespread across Africa, whose climatic niche indicates adaptive divergence along rainfall gradients, probably boosted by past climate fluctuations.
Faidherbia albida is a monospecific taxon (POWO - Plants of the World Online, 2021) and is a potentially good model to test possible environmental correlations among different geographic areas, using climatic modelling and analyses of land use and land cover (LULC) changes. The distribution of this tree has been successfully modeled using MAXENT, in central Senegal, although with a relatively low AUC (0.64), where a relationship between tree diversity and human activities was found (Ndao et al., 2022).
Most of native and endemic trees of Cabo Verde have seen their distribution ranges reduced (Varela et al., 2022), most likely due to human action, while they could play a decisive role in a more sustainable development, further considering the possible effects of future climate change. Therefore, to support the definition of sounder land management actions devoted to the valorization of the ecosystem services provided by those taxa, we need a better understanding of the factors affecting their distribution. In this context, this study aimed to estimate the current distribution and potential shifts in suitable areas for F. albida under climate change, using species distribution models. We used SDMs to determine the climatic niche of F. albida, both in mainland Africa and in Cabo Verde. We then projected its potential distribution in Cabo Verde based on the current climatic conditions and according to the future climate change scenarios. Finally, we overlapped the present potential distribution of F. albida in Cabo Verde with LULC data to identify possible constraints. We developed our distribution models also including data from mainland Africa, since we wanted to determine, as accurately as possible, the climatic niche of the study species. Working only with distribution and climatic data from Cabo Verde could potentially lead to a biased circumscription of the climatic niche, since F. albida distribution range in Cabo Verde is much more limited than in mainland Africa.
We think that this first step constitutes the baseline for the development of innovative research and management actions that will allow the valorization of F. albida in terms of biodiversity preservation, soil conservation, carbon accumulation and human use in Cabo Verde.
2. Materials and methods
2.1. Study area
The archipelago of Cabo Verde (Figure 1) lies between latitudes 14–18°N and longitudes 22–26°W and is included in the African Sahelian arid and semi-arid climatic region, the largest semi-arid region of the world, which extends across Africa from the Atlantic Ocean to the Indian Ocean (Varela-Lopes and Molion, 2014; Neto et al., 2020). The hydrological resources are scarce, with an average annual precipitation of 352 mm/year from 1990 to 2016, well below the average of 653 mm/year recorded in the African continent for the same period (CCKP, 2019).
The islands of Cabo Verde were uninhabited until the 15th century, and their climate, tropical and drier than in the other Macaronesian archipelagos, did not encourage the discovery of its flora (Romeiras et al., 2020). The first field expeditions made by naturalists only occurred at the end of the 18th century (more details in Romeiras et al., 2014). The broad scale patterns of plant richness are mainly affected by climate and topography, as altitude and exposure to northeast trade winds lead to contrasting weather conditions (Duarte et al., 2008). The northern (i.e., São Nicolau, São Vicente, and Santo Antão) and southern islands (i.e., Brava, Fogo, and Santiago) are characterized by high mountains, offering a wide range of habitats over relatively short distances, whereas the eastern islands (i.e., Maio, Boavista, and Sal) are lower and drier (Neto et al., 2020).
2.2. Study species
The apple-ring acacia or espinheiro-branco (common name in Cabo Verde) Faidherbia albida (Figures 1B,C; synonyms: Acacia caboverdeana Rivas Mart., Lousã, J.C. Costa & Maria C. Duarte; Acacia albida Delile: see Rivas-Martínez et al., 2017) is considered native to Cabo Verde (Romeiras et al., 2016, 2020). Segregated from the genus Acacia by Chevalier in 1934 and confirmed by Luckow et al. (2003) based on molecular studies, this genus belongs to Ingeae and not to Acacieae. It is a leguminous tree species with an important role in West African agroforestry areas (Vandenbeldt, 1992; Lu et al., 2022). In addition, it contributes to symbiotic nitrogen fixation and provides a variety of ecosystem services, such as nutrient recycling and carbon sequestration, among others (Lu et al., 2022). Faidherbia albida has a unique phenology, as compared with other tree species since it is leafless during the wet season and grows its leaves and pods during the dry season, which reduces the competition for water, light, and nutrients with associated crops during the wet season (Vandenbeldt, 1992; Hadgu et al., 2009; Lu et al., 2022).
2.3. Occurrence data
The data on the occurrence of the study species (see Figure 1A) were collected from the following sources:
- Cabo Verde data were obtained from herbarium records (see Supplementary Table with the list of herbarium specimens); and field surveys undertaken by MR that also originated herbarium material;
- For mainland Africa, 1,354 records from 55 published datasets were available in Global Biodiversity Information Facility (GBIF.org, 2021; https://doi.org/10.15468/dl.rxn1jv), but several occurrence points in very specific areas (e.g., rivers, and seas) were eliminated to avoid possible outliers.
The precise geographic coordinates of each record were obtained, with an error below 10 m, and all the considered occurrences of F. albida for Cabo Verde (34) and for mainland Africa (442) were organized into a database.
2.4. Environmental variables
The bioclimatic variables for continental Africa (Supplementary data 1, 2) were downloaded from the WorldClim v2.0 dataset1 at a resolution of 30 arc-seconds (Fick and Hijmans, 2017). The bioclimatic variables available in WorldClim and CCAFS2 have been extensively used in plant distribution modelling (Deblauwe et al., 2016; Booth, 2018; Perrin et al., 2020). To investigate the relationship between variables and to avoid excessive collinearity, we used the Variance Inflation Factor (VIF; Newman et al., 2010). The predictors with the highest VIF values were initially eliminated, and new VIF scores were calculated for the remaining predictors; this procedure was repeated until all VIF scores were less than 10. This procedure was conducted in R v3.6.0 (R Development Core Team, 2020). The data layers were prepared by using the QGIS software 2.18.20 (QGIS Development Team, 2021). All variables were projected into the same Geographic Coordinate System (WGS84), selecting the method of the nearest neighbor resampling. This approach followed Silva et al. (2019) and Varela et al. (2022).
2.5. Future climate
To examine the effects of climate change on the distribution of Faidherbia albida, potential distributions were projected using the 5th assessment report (AR5) of the Intergovernmental Panel on Climate Change (IPCC), available at the International Center for Tropical Agriculture website (see http://www.ccafs-climate.org). The AR5 was chosen as the most comprehensive assessment of climate change and as a reliable basis for policymaking (Symon, 2013). The AR5’s underlying scenarios of human influence, dubbed Representative Concentration Pathways (RCPs), are expressed in terms of greenhouse gas concentrations. Each RCP implies a different amount of human-driven climate change, resulting in a different amount of extra heat energy being stored in the Earth system due to greenhouse gas emissions (Table 1). These scenarios were developed using assumptions concerning economic growth, technology choices, and land use (Symon, 2013). In this study, we followed two scenarios (RCP 4.5 and RCP 8.5) described in Table 1.
2.6. Modelling
2.6.1. Modelling framework and algorithms
Implications of climate change on the distribution of Faidherbia albida were predicted through a modelling approach - BIOMOD (BIOdiversity MODelling; “biomod2”; Thuiller et al., 2020). BIOMOD is a freeware, open-source package implemented in R version 3.6.1 that aims to maximize the predictive accuracy of current species distributions and the reliability of future potential deliveries, using different types of statistical modelling methods (Thuiller, 2003; R Development Core Team, 2020). We followed the approach implemented by Silva et al. (2019, 2021), and Varela et al. (2022) and the main steps are summarized in the Figure 2.
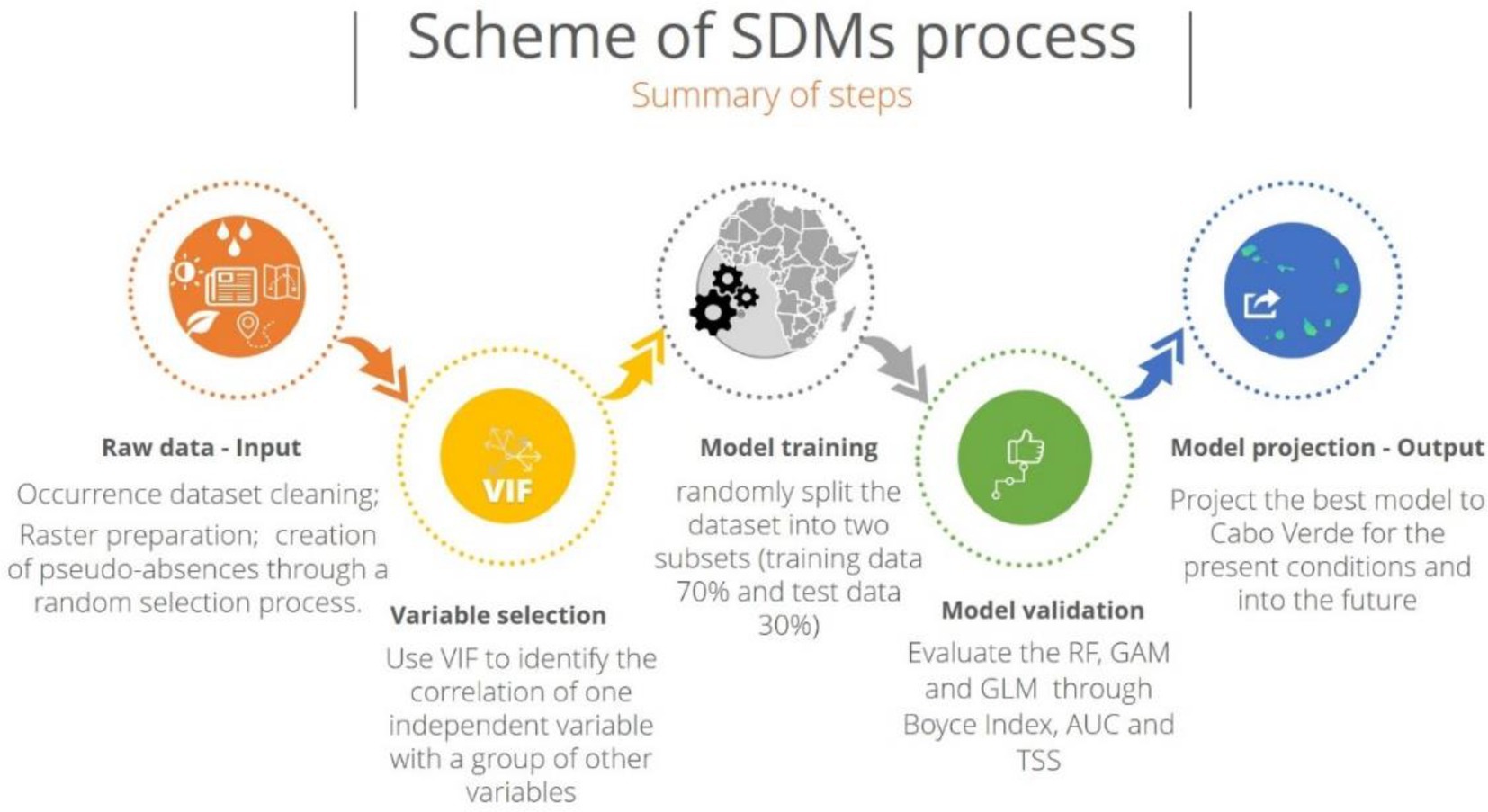
Figure 2. General scheme of the modelling framework, based on Silva et al. (2019, 2021) and on Varela et al. (2022).
We used a total of three different SDM algorithms implemented in the BIOMOD2 package: Generalized Linear Models (GLMs; Guisan et al., 2002; Emptage and Dobson, 2008), Generalized Additive Models (GAM; Guisan et al., 2002; Rigby et al., 2005), and one machine learning method, Random Forest (RF; Breiman, 2001). RF is a regression tree created by using random feature selection in tree induction and bootstrap samples of the training data (Breiman, 2001). GLMs and GAM use a link function to establish a relationship between the mean of the response variable and the explanatory variables (Guisan et al., 2002; Rigby et al., 2005). For further methodological details about the three SDM algorithms implemented, see Silva et al. (2019, 2021) and Varela et al. (2022).
The use of RF in SDMs has been demonstrated to be robust and stable when handling multicategory or binary abundance information, e.g., presence and absence data (Hegel et al., 2010). Comparisons of different SDMs revealed that presence-absence models perform better than presence-only models (Elith et al., 2006). Because absence points were not included in our initial data set, we generated pseudo-absences through a random selection process. This technique involves randomly selecting pseudo-absence points from the background data across the entire study area. The number of pseudo-absences used for all the algorithms was equal to the number of occurrences because the predictive accuracy increased when the number of pseudo-absences was approximately equal to the number of presences (Barbet-Massin et al., 2012). We used the “random Points” function in the R package “dismo” to reduce projection bias (Hijmans et al., 2020).
The model training was performed using the whole African territory. Cross-validation was used because a validation set was not available, thus we randomly split the dataset into two subsets (training data 70% and test data 30%). The first subset was used to fit the model, and the second subset was used to evaluate the model’s performance, before making predictions and comparing them to the expected values. This procedure was repeated 10 times for each modelling algorithm, as suggested in the BIOMOD2 package and applied in previous research (Silva et al., 2019, 2021; Varela et al., 2022), allowing the calculation of a mean and a standard deviation for the three evaluation parameters and for each modelling approach.
The Boyce Index, the area under the curve (AUC) of the receiver-operating characteristic (ROC), and the true skill statistic (TSS) were used to evaluate the models (Boyce et al., 2002; Allouche et al., 2006; Pearce and Boyce, 2006; Singer et al., 2017). Both evaluation indicators were calculated using the proportion of “sensitivity” (correctly predicted presences) and “specificity” (correctly predicted absences). The models’ performance to predict the currently suitable area was good, with TSS values ranging from 0.64 (GLM) to 0.92 (RF), AUC values ranging from 0.63 (GAM) to 0.99 (RF), and Boyce Index values ranging from 0.43 (GLM) to 0.84 (RF).
The best models for the current distribution of Faidherbia albida were projected for the present conditions and for two different future time slices (2050 and 2080) using the respective functions of BIOMOD 2 and the predicted future climate conditions from the IPCC’s 5 assessment taken from the International Centre for Tropical Agriculture website (see http://www.ccafs-climate.org/).
2.6.2. Land use and land cover probability maps
Taking into account the effect of Land Use and Land Cover (LULC) on habitat availability and suitability, as reported by Fahrig (2003), Zhang et al. (2017), and Wang et al. (2019), we developed maps of LULC probabilities. For this purpose, we used the Cabo Verde and West Africa LULC’s maps (see Figure 3; Supplementary data 3) provided by the United States Geological Survey (USGS; CILSS, 2016). These maps are based on visual interpretation of Advanced Spaceborne Thermal Emission and Reflection Radiometer (ASTER), which characterizes the landscapes at a resolution of 500 m and 2 km, for Cabo Verde and West Africa, respectively, between 2000 and 2013.
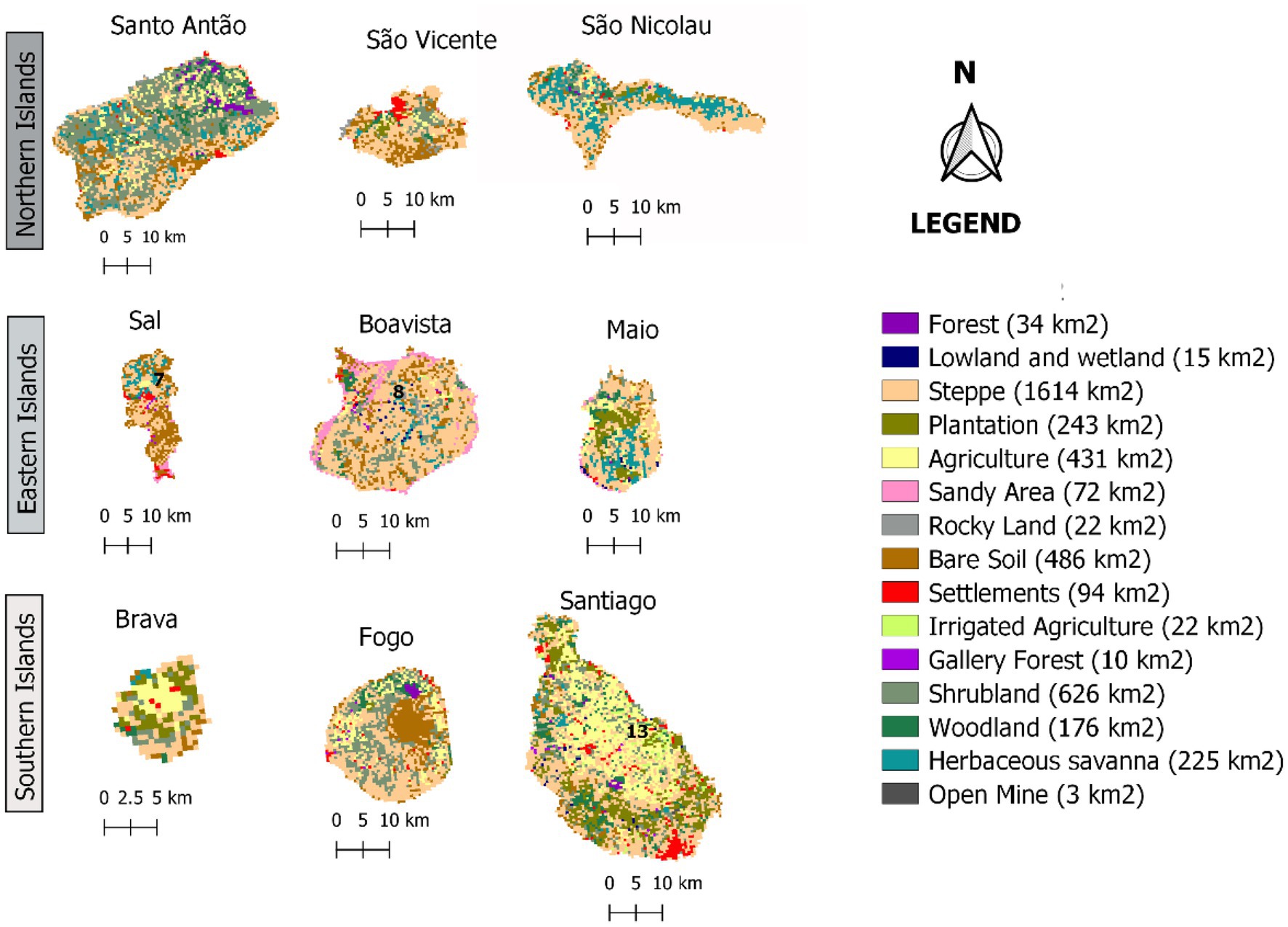
Figure 3. Land Use and Land Cover maps of the archipelago’s nine inhabited islands in 2013 (CILSS, 2016).
Based on the LULC data for Cabo Verde, on a sample of 342 cells in Cabo Verde and West Africa covering the different types of LULC, and on the occurrences and absences of Faidherbia albida in Cabo Verde per type of LULC category, a LULC Index was calculated using two different approaches (Supplementary data 4):
a) the ratio between the percentage of occurrences in a given LULC category and the percentage of Area of that LULC category:
Occurrences for for each category, .
b) the Bayes Theorem with the following calculations:
= , for each LULC category, , where is the probability of finding Faidherbia albida in Cabo Verde, based on its frequency (percentage of 1 km2 cells with the species), is the probability of not finding Faidherbia albida in Cabo Verde, based on its absence (percentage of 1 km2 cells without the species), is the probability of finding the category in the cells occupied by this species in Cabo Verde, is the probability of finding the category in the cells not occupied by this species in Cabo Verde, and P(FA| ) is the resulting probability of finding this species in a cell with the category.
Since both approaches fully agreed when we ranked the LULC categories, we then attributed the final values of the LULC Index to each category, as shown in Table 2. When producing the distribution map adjusted to the adequacy of each LULC category, for each cell in the map, we multiplied the respective LULC Index by the present habitat suitability value derived from SDMs.
According to the LULC index and human pressure, the original LULC was recategorized as Suitability Index, according to the criteria displayed in Table 2.
3. Results
3.1. Climatic niche of Faidherbia albida
The Table 3 summarizes the climatic conditions under which the studied tree species currently occurs, and Figure 4 depicts the descriptive statistic values of the bioclimatic variables used in modelling.
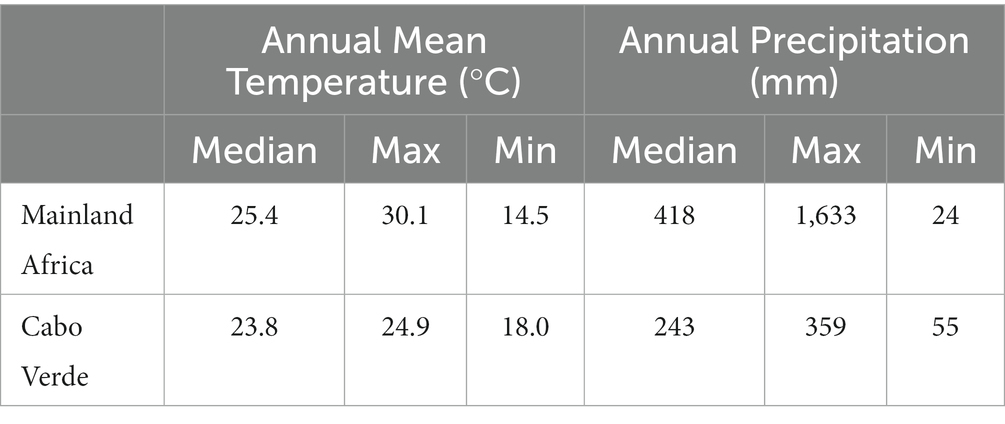
Table 3. Annual mean temperature and precipitation for the current habitat conditions of Faidherbia albida in mainland Africa and Cabo Verde.
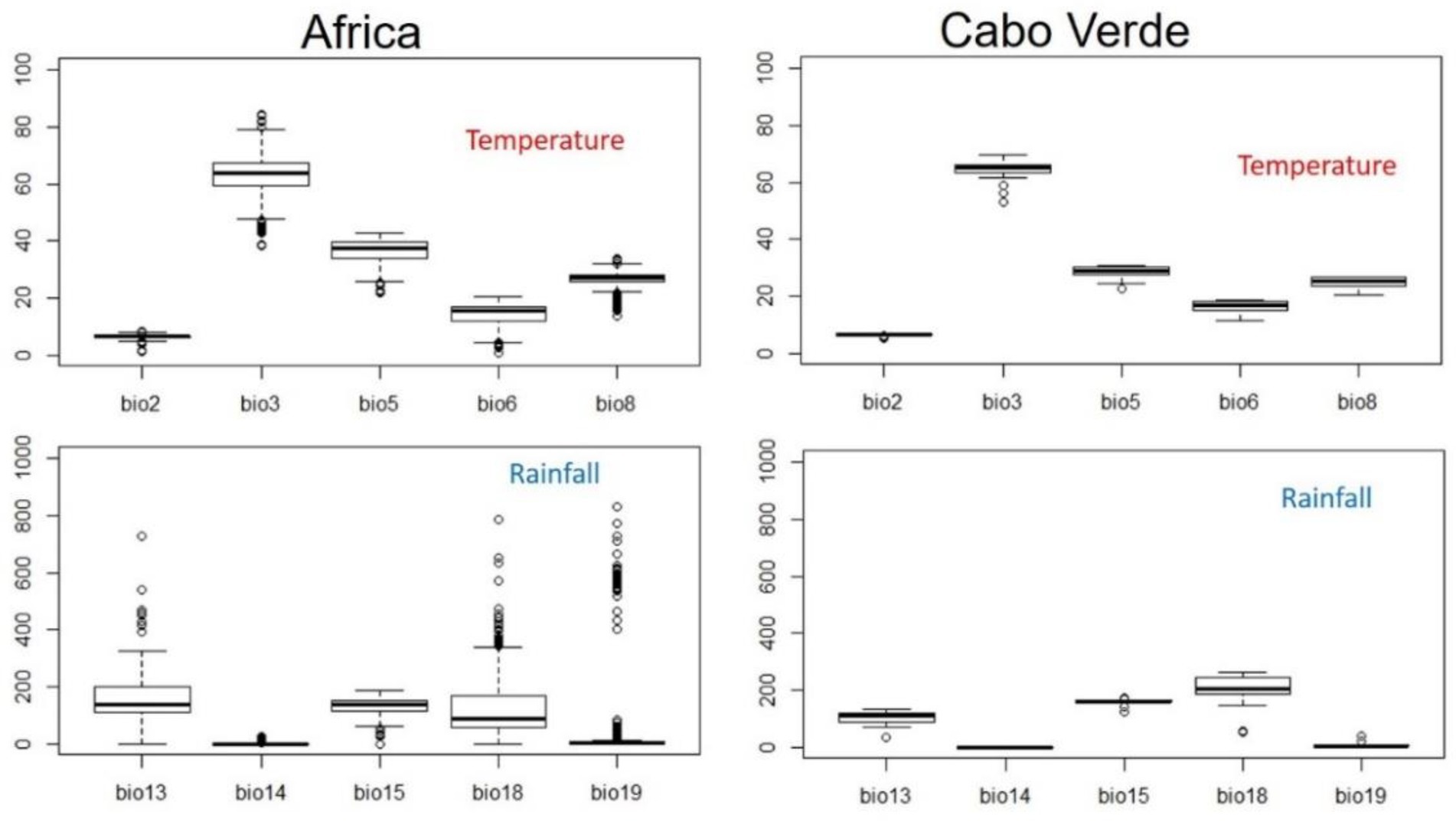
Figure 4. Descriptive values of bioclimatic variables used to model the distribution of Faidherbia albida in Cabo Verde, using data from mainland Africa to estimate its climatic niche. BIO2 (Mean Diurnal Range), BIO3 (Isothermality), BIO5 (Max Temperature of Warmest Month), BIO6 (Min Temperature of Coldest Month), BIO8 (Mean Temperature of Wettest Quarter), BIO13 (Precipitation of Wettest Month), BIO14 (Precipitation of Driest Month), BIO15 (Precipitation Seasonality), BIO18 (Precipitation of Warmest Quarter), BIO19 (Precipitation of Coldest Quarter).
As expected, in continental Africa Faidherbia albida occurs under a much wider range of climatic conditions than in Cabo Verde. As a consequence, there is only a moderate difference in average values of bioclimatic variables between the two studied regions.
In terms of climate change scenarios, under RCP 4.5 in 2080 (Supplementary data 1), the average annual mean temperature in the areas currently occupied by F. albida will rise by 1.5°C. The mean values of some bioclimatic variables will increase, namely BIO6 (Min Temperature of Coldest Month), BIO9 (Mean Temperature of Driest Quarter), and BIO13 (Precipitation of Wettest Month). In contrast, BIO3 (Isothermality), BIO13 (Precipitation of Wettest Month), and BIO15 (Precipitation Seasonality) are expected to decrease.
3.2. Variable and model selection
Out of 19 bioclimatic variables available for modelling, nine were excluded after VIF analysis, and the remainder were used as model predictors (Table 4).
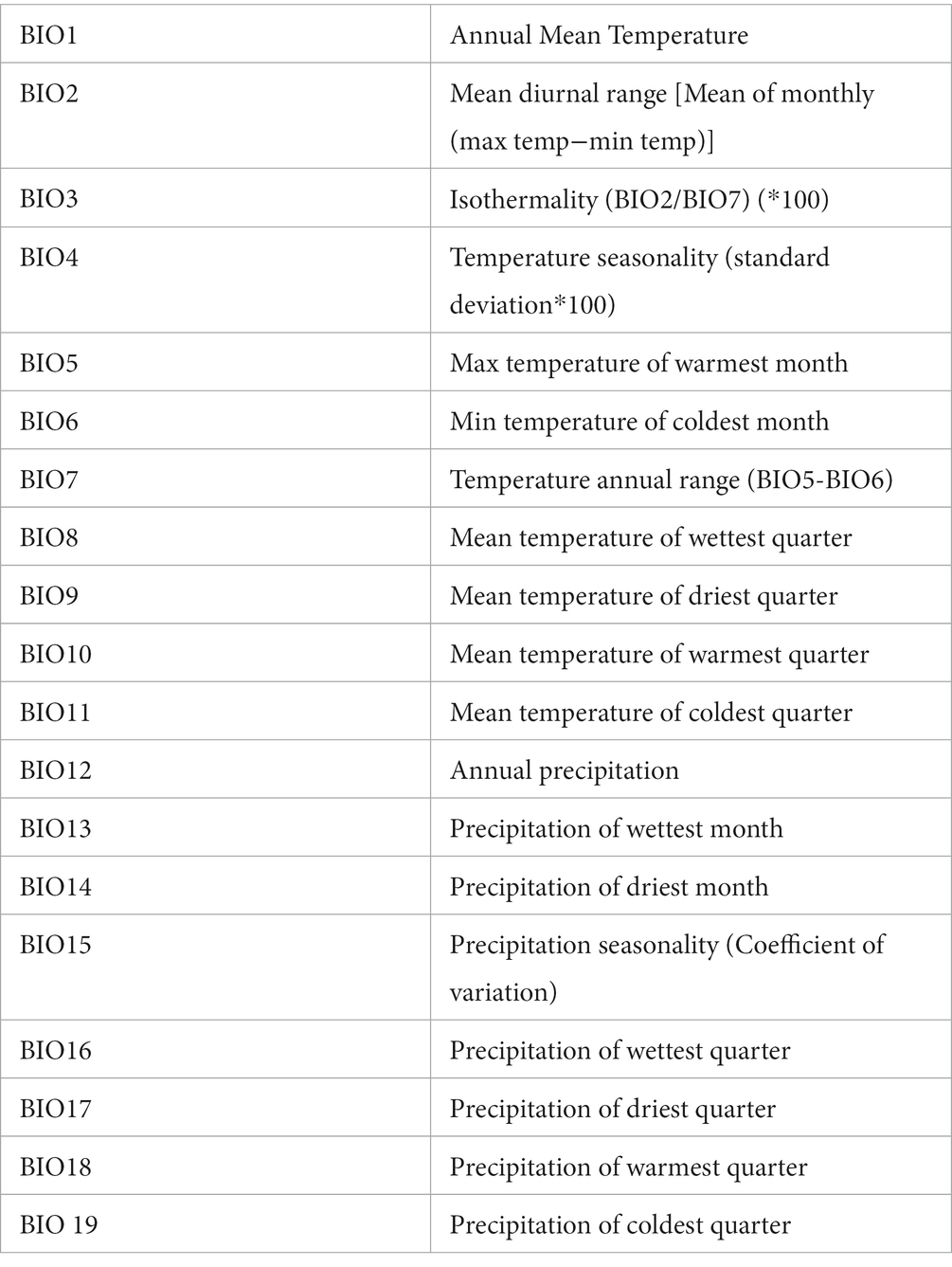
Table 4. Environmental variables used to predict Faidherbia albida distribution in Cabo Verde; non-highlighted variables were removed after applying Variance Inflation Factor (VIF) analysis to reduce collinearity.
3.3. Model evaluation
Random Forest (RF) performed better than GLM and GAM (Table 5) to predict Faidherbia albida suitable distribution under present and future climate scenarios. TSS values ranged from 0.55 (GLM) to 0.92 (RF), and AUC values ranged from 0.64 (GLM) to 0.99 (RF).
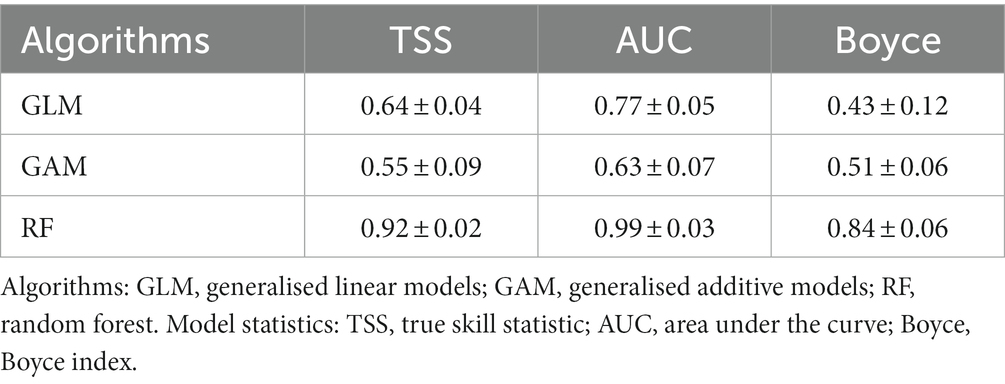
Table 5. Statistics used to evaluate the performance of climatic models explaining the presence/absence of Faidherbia albida in Cabo Verde and Africa Mainland.
The variables with more impact on the distribution of F. albida were BIO15, BIO13, BIO18, BIO6 (Figure 5).
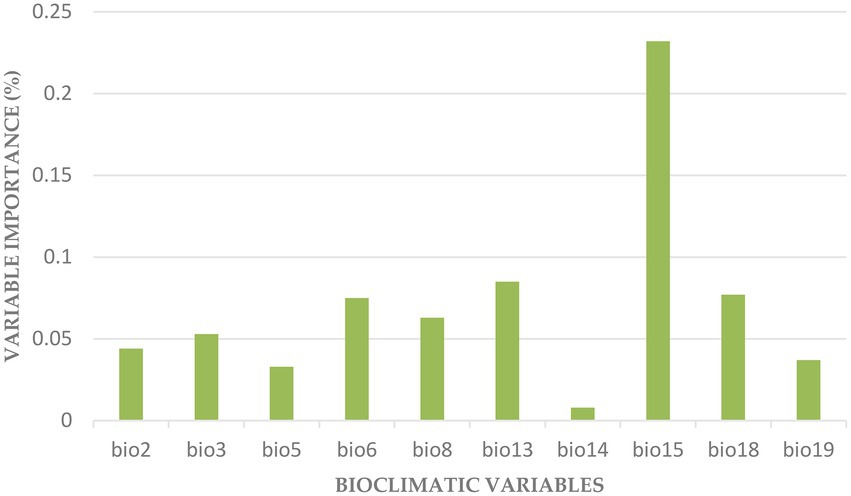
Figure 5. Importance of the bioclimatic variables in the final model: BIO2 (Mean Diurnal Range), BIO3 (Isothermality), BIO5 (Max Temperature of Warmest Month), BIO6 (Min Temperature of Coldest Month), BIO8 (Mean Temperature of Wettest Quarter), BIO13 (Precipitation of Wettest Month), BIO14 (Precipitation of Driest Month), BIO15 (Precipitation Seasonality), BIO18 (Precipitation of Warmest Quarter), and BIO19 (Precipitation of Coldest Quarter).
3.4. Model projections – Present climate
Our results show that under current climatic conditions (see Figure 4) about 89% of the African territory is unsuitable for Faidherbia albida. Most of the appropriate area for the occurrence of this species is the Sahel region, by the southern edge of the Sahara Desert, at the same latitude as Cabo Verde, according to our occurrences map (Figure 6).
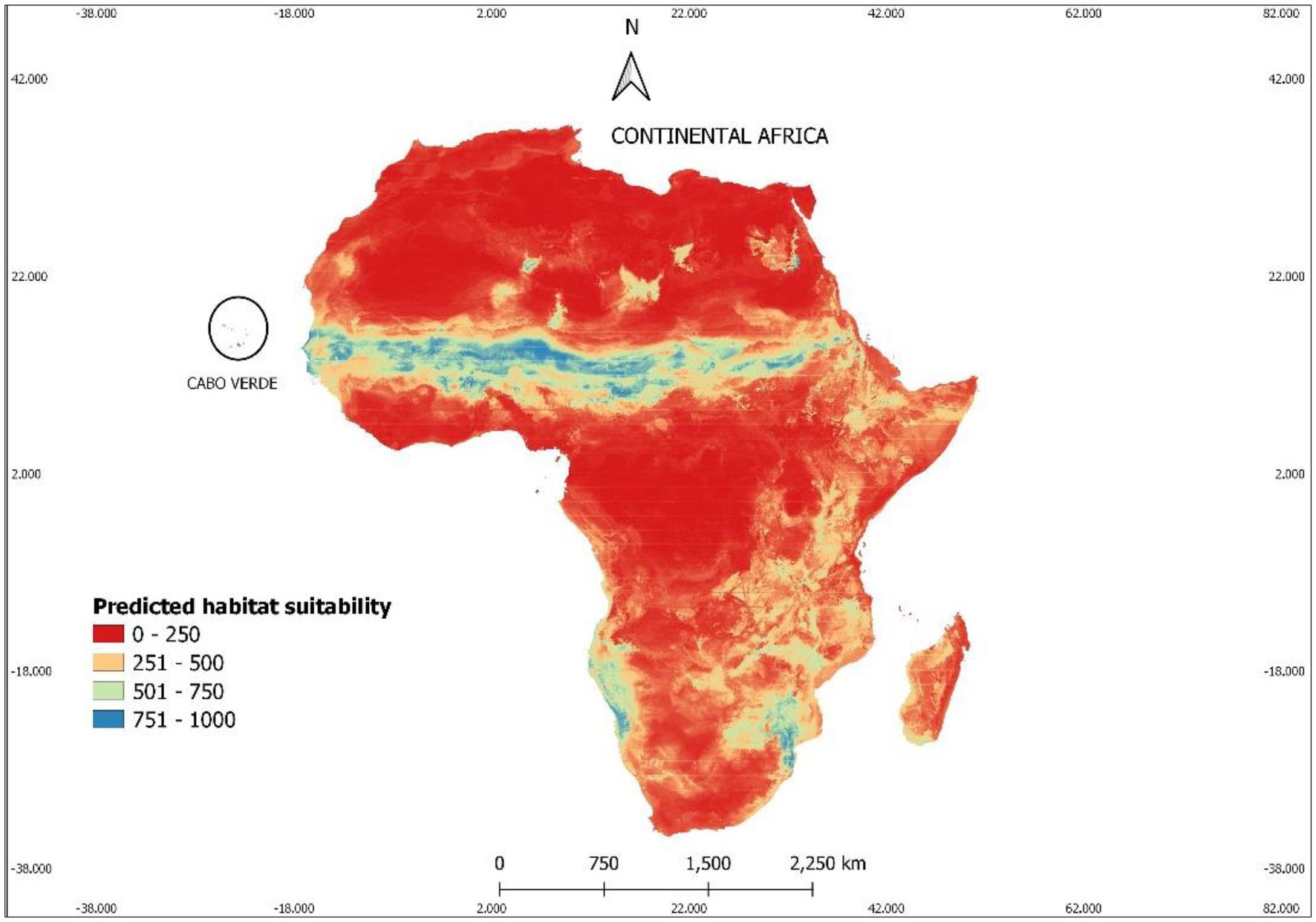
Figure 6. The current suitable area for Faidherbia albida in Africa, according to the results of climatic modelling. Suitable areas correspond to probabilities of occurrence above 50%. The colors indicate predicted suitable habitats ranging between red (low) and blue (high). Habitat suitability values in the 0–1,000 format, to facilitate reading.
When only bioclimatic variables were used to determine habitat suitability, almost the entire archipelago appeared suitable for the occurrence of the studied species, with 43% corresponding to values above 750, and 49% with values above 500 (Figure 6). However, most of the area of some islands (namely Santo Antão, Sal, and Boavista) showed low habitat suitability (Figure 7).
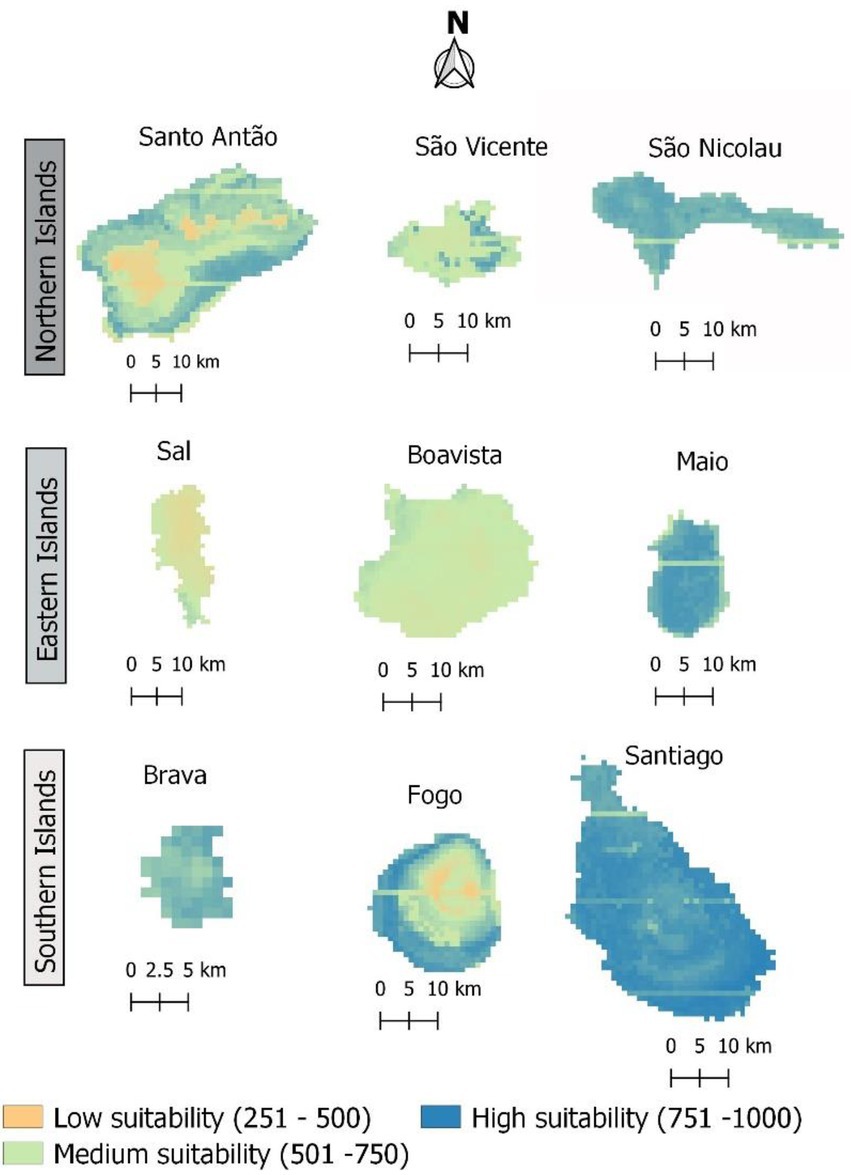
Figure 7. Current suitable area for Faidherbia albida in Cabo Verde. Habitat suitability values are provided in the 0–1,000 format, to facilitate reading.
3.5. LULC effect on the climate-model projections
Despite its small size, the archipelago of Cabo Verde has an extremely diverse landscape. However, its arid steppe regions cover approximately 1,614 km2, accounting for nearly 40% of the total land area. Additionally, the highest number of occurrences of F. albida is in steppe areas, but the lowlands and wetlands have the highest density (number of occurrences per km2).
In Western Africa, the savanna hosts the highest frequency of F. albida occurrences. However, during the mid-20th century, afforestation practices in Cabo Verde promoted plantations of fast-growing trees, such as Prosopis juliflora, which affected the vegetation structure and composition in these islands. Figure 8 shows the present habitat suitability maps for Cabo Verde, after overlaying LULC constraints to the climatic model, which leads to a possible reduction in high habitat suitability for F. albida, from 49% to less than 20% of the territory.
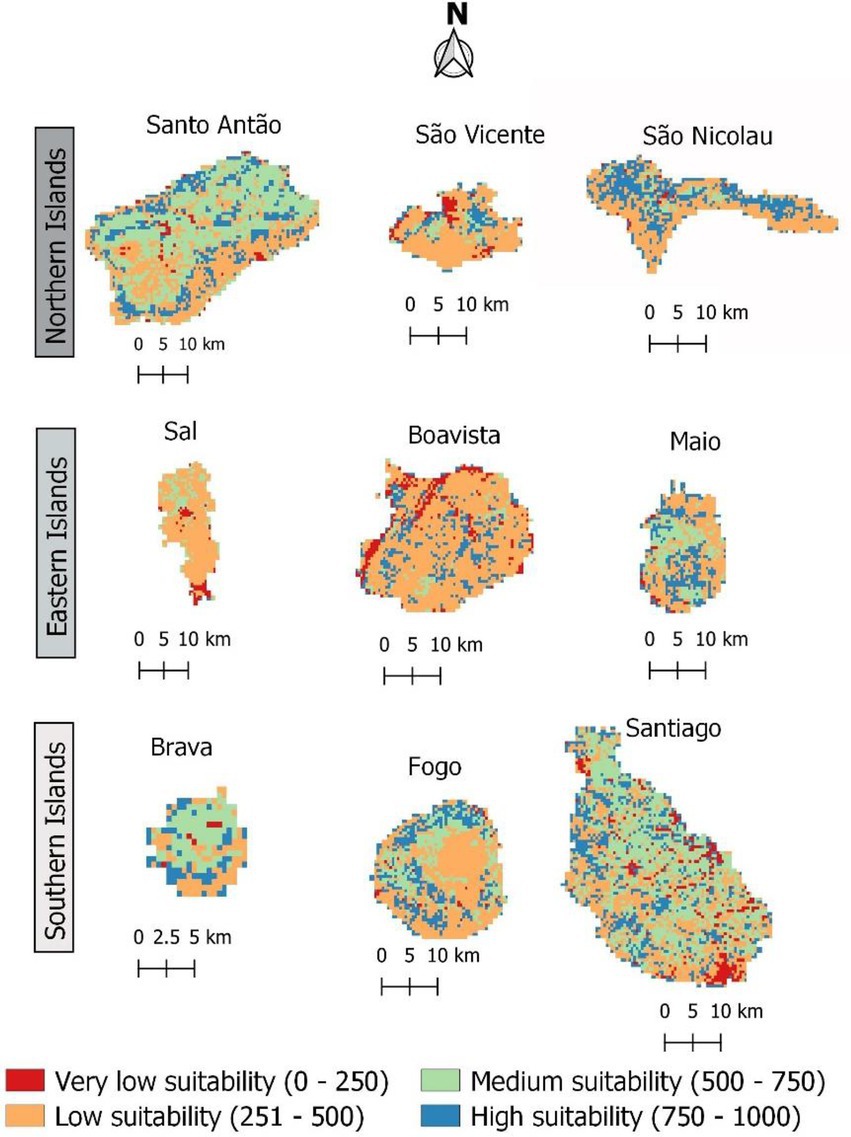
Figure 8. The current suitable area for Faidherbia albida in Cabo Verde, according to the results of climatic modelling and the constraints derived from different LULC categories. Suitable areas are those corresponding to probabilities of occurrence above 50%. Habitat suitability values are shown in the format 0–1,000 to facilitate reading. The colors indicate predicted suitable habitats ranging from red (low) to blue (high).
3.6. Model projections – Future climate
In both scenarios, according to model predictions, the extension of habitat suitable for Faidherbia albida in Cabo Verde is expected to slightly decrease in the future, and with almost identical patterns (Figure 9; Table 6).
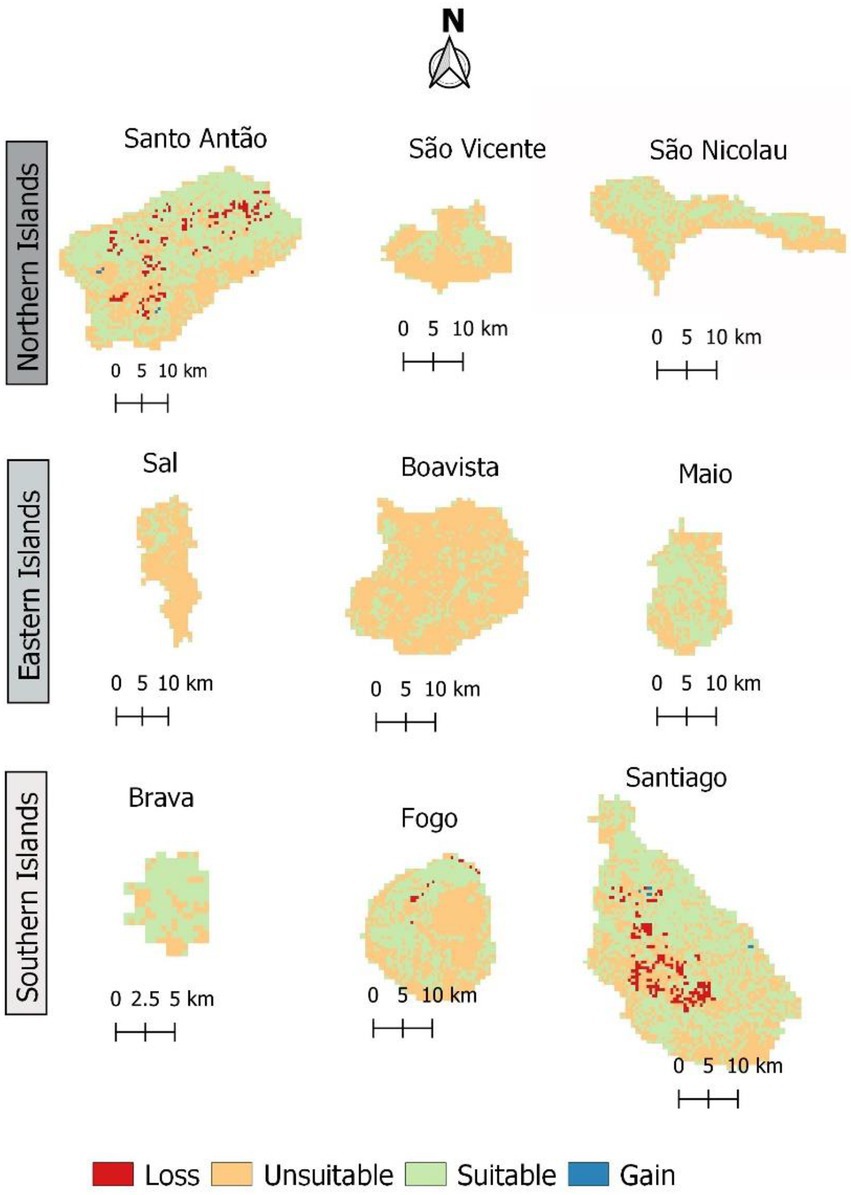
Figure 9. Change in habitat suitability for Faidherbia albida in Cabo Verde, by 2080 under RCP 4.5. Loss indicates areas where habitat suitability is expected to decline. Gain shows areas where habitat suitability is expected to increase.
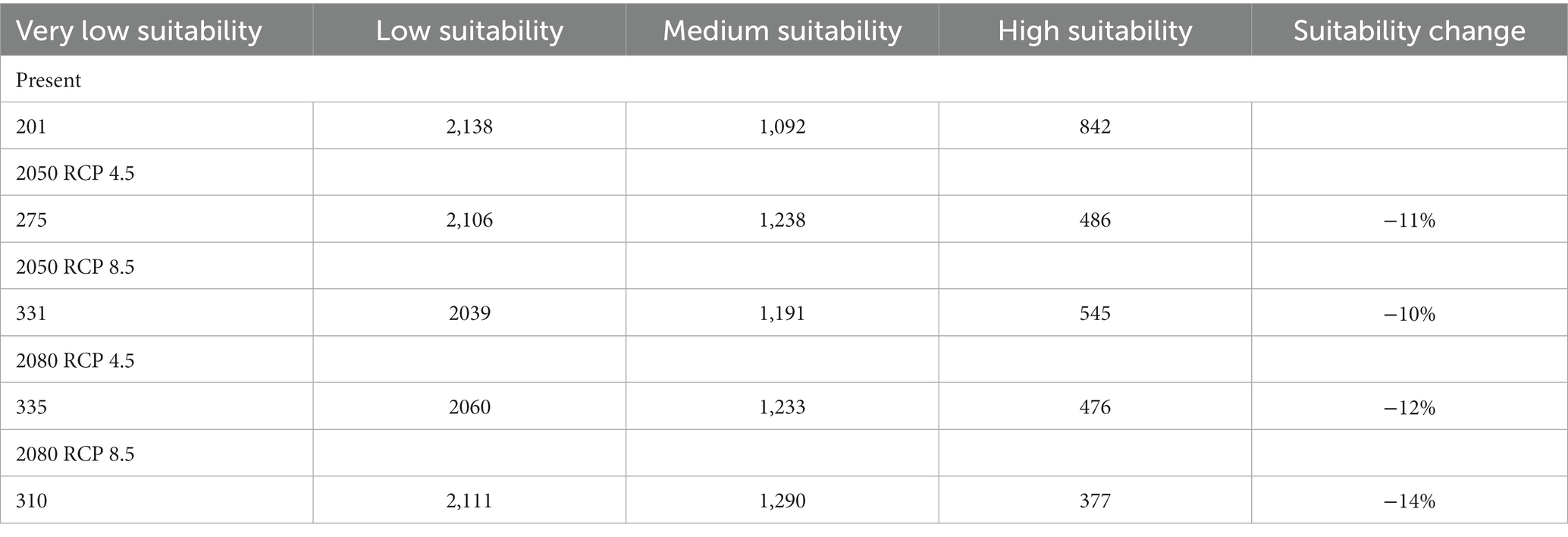
Table 6. Suitable habitat in km2 and variation in percentage, for the tree species Faidherbia albida in Cabo Verde, under two climate change scenarios (RCP 4.5 and RCP 8.5), based on climatic modelling.
By 2050, under RCP 4.5, the suitable area will decrease from 1934 to 1716 km2 – about 11%. Under all scenarios, the suitable area (i.e., medium and high suitability) will remain high, corresponding to more than 30% of the territory. In the most pessimistic scenario (RCP 8.5), the expected decrease of suitable habitats is 13%. In Cabo Verde, under this scenario, the Annual Mean Temperature (BIO1) is expected to increase from 25.9°C (sd = 2.30) to a maximum of 29.6°C (sd = 2.8), a difference of 4°C. Annual Precipitation (BIO12) is expected to increase from 598 to 604 mm by 2080.
Gains occur when species colonize new areas, while species losses occur when habitat suitability is reduced in the future. Suitable habitat areas are expected to decrease mainly in Santo Antão and Santiago islands. The gain and loss maps are presented in Figure 9. For the year 2080, under scenario RCP 4.5, a slight habitat loss is expected. The high suitability category will greatly decrease in all scenarios, but the medium suitability area will increase under all climate change scenarios.
4. Discussion
Modelling the bioclimatic niche of Faidherbia albida – one of the few native tree species of Cabo Verde, provided new data to identify the environmental and anthropogenic constraints that might have affected their distribution in the past, and restrain future range. Our results enabling the establishment of priorities in the management and future afforestation activities, which are extremely important in Cabo Verde, one of the most vulnerable West African countries. SDMs are, therefore, an invaluable tool for decision-makers because they can improve the conservation strategies to be applied in areas deeply affected by climate change.
4.1. Climate niche, past, present, and future distributions
Concerning the global model evaluation, the RF algorithm produced the highest-quality models. Numerous studies have demonstrated that RF is excellent in predicting species distribution patterns (Ren-Yan et al., 2014; Silva et al., 2019, 2021; Varela et al., 2022). The highest quality of RF algorithm was confirmed not only through TSS and AUC (Pearce and Boyce, 2006) but also by analyzing the continuous Boyce index and the shape of the corresponding curves, which had a low standard deviation and ascending, nearly linear curves (Boyce et al., 2002; Marmion et al., 2009; Costa et al., 2013). Furthermore, Varela et al. (2022) concluded that RF was the best model to estimate the climatic niche of endemic tree species in Cabo Verde. According to the values of those parameters, we obtained better model fits than those previously obtained when modelling F. albida in Senegal (Ndao et al., 2022).
Faidherbia albida thrives under a broad variety of climatic conditions. For instance, in West Africa, it can occur in a desert environment where the mean annual rainfall is only 24 mm, and the mean annual temperature is 14.5°C (see Table 2). It also occurs in humid tropical areas, with 1,633 mm of annual precipitation and a mean annual temperature of 30°C. In Cabo Verde, it occurs under a wide range of climatic conditions as well. However, in locations with limited rainfall, F. albida requires episodic downpours of rain to grow (Barnes and Fagg, 2003).
According to our modelling results, the Cabo Verde climate seems appropriate for F. albida. However, its present distribution does not reflect its potential climatic niche, which suggests that factors such as land-use changes (USGS, 2022) and the widespread expansion of non-indigenous trees (e.g., Prosopis spp., Cienciala et al., 2013) might have limited the present occurrence of this species in the archipelago.
A significant difference exists between endemic tree species and Faidherbia albida in terms of the impact of the archipelago’s climate on their distribution. Varela et al. (2022) examined the effect of climate change on the distribution of the threatened endemics Dracaena draco subsp. caboverdeana, Phoenix atlantica and Sideroxylon marginatum, and found it will significantly impact their distribution. For instance, less than 5% of the territory is highly suitable for Dracaena draco subsp. caboverdeana. For F. albida, currently, roughly 800 km2 correspond to highly suitable land, but by 2080 this area will be less than 400 km2, an expected reduction of 50%, and corresponding to 9% of the territory. The medium suitable area, on the other hand, will increase, thus globally reducing the area by only 10–14%. Even though the direct consequences of climate change indicated by the models are not severe, it is vital to consider all the impacts on the biosphere on a global scale, particularly in Africa, where climate change is expected to be more severe than in other parts of the globe (Fick and Hijmans, 2017; Noulèkoun et al., 2017; IPCC, 2018a,b, 2022).
In Cabo Verde, the island of Santiago is the most suitable one for F. albida, since it is the largest and has the most favorable soil and climatic conditions, thus explaining the highest concentration of native tree species on this island. Indeed, in comparison to Sal, Boavista, and Maio, the mountainous Santo Antão, São Nicolau, Santiago, Fogo, and Brava, all with peaks over 1,000 meters, have the most suitable areas due to their more productive soil, cooler temperatures, and higher precipitation. According to Neto et al. (2020), climate and topography play a significant role in shaping the diversity of plant communities in Cabo Verde islands, particularly in northeast slopes of mountain areas.
4.2. Effect of LULC
The climatic conditions of Cabo Verde were shown to be adequate for Faidherbia albida, but, the present distribution of this tree is restricted to fairly small patches, which does not reflect its potential climatic niche. The land use of Cabo Verde underwent significant changes during human settlement (Duarte et al., 2022). Mainly intensive agricultural practices, and many introduced species have cleared a large portion of native tree populations (Monteiro et al., 2020; Varela et al., 2022). In many areas, this led to soil erosion, and by the early twentieth century the islands were severely degraded (USGS, 2022).
The anthropogenic pressures associated with land-use and land-cover changes (e.g., fire, wood extraction, introduction of herbivores, and agricultural practices) have resulted in a shift toward sparser woody plant vegetation, after more than 500 years of human settlement in Cabo Verde (Romeiras et al., 2016; Norder et al., 2020). In the 1970s, exotic trees were widely introduced, but, until then, the only source of firewood were native trees, which may have contributed to reduce their numbers across the archipelago (Varela et al., 2022). A similar situation to that of F. albida was found for the endemic trees of Cabo Verde, which are presently very rare, most likely due to land use changes and native vegetation clearing in the past (see Varela et al., 2022).
According to our analysis, the most suitable land-use types for F. albida are, in descending order: lowlands and wetlands, rocky land, agriculture, irrigated agriculture, gallery forest, and forest. The least suitable are open mines and settlements, followed by shrubland, woodland, sandy area, savanna, grassland, bare soil, plantation, and steppe. The less suitable areas are characterized by the absence or very low occurrence of F. albida and low or no vegetation or tree cover. The most appropriate areas are characterized by the presence of F. albida and tree cover.
In the Central Rift Valley of Ethiopia, it was found that the limited seed source, caused by excessive pruning, was the main constraint for recruitment, and that an appropriate land management policy to ensure adequate seed production would avert current trends in decline of F. albida population (Sida et al., 2018a). A clear indication of how land use and land management practices might have been responsible for a similar decline in Cabo Verde.
Historically, the land-use change in Cabo Verde has been characterized as a transition from a dry but “well-wooded” savanna with “great quantity of grass,” and “streamlets of water,” at the time of human settlement, to a near desert landscape, especially at the lower altitudes, as a direct consequence of overexploitation of the vegetation-cover by humans and goats (see Lindskog and Delaite, 1996). Specific research devoted to that archipelago has shown that several areas are highly vulnerable to population growth, intensification of agricultural land use and increasing tourism (Olehowski et al., 2008), due to high slopes, friable geological substrates, and precipitation (higher at steeper locations). Moreover, Palaeoecological studies (Castilla-Beltrán et al., 2019) recently showed the anthropization of the highlands of Santo Antão Island, Cabo Verde. That study revealed that from ca. 350 to 250 cal yr. BP, human-induced environmental changes, such as the introduction of new pant taxa and of alien herbivores, and vegetation clearing for agricultural activities, has led to overgrazing, vegetation burning, and soil erosion. This strongly suggests that the distribution of F. albida in Cabo Verde was probably more widespread in the past, what would agree with the results of our modelling analyses, based on its climatic niche both for Africa and Cabo Verde, indicating a much wider potential distribution in the archipelago when compared to its presently observed occurrence.
The combined effects of land use and climate change have been considered the greatest threat to global biodiversity (García-Valdés et al., 2015; Wang et al., 2019; Chen and Leites, 2020). Thus, climate and land-use changes are expected to substantially alter future plant species distributions leading to higher extinction rates. Landscape composition and structure can impose constraints on habitat availability and suitability (Fahrig, 2003; Zhang et al., 2017; Wang et al., 2019). Therefore, the LULC probability maps that we developed seem to be a useful framework in future modelling to better understand the role of LULC in constraining the potential distributions mainly defined by the climatic niche.
4.3. Valorization of Faidherbia albida ecosystem services
Given our results, areas that are climatically suitable for Faidherbia albida and where LULC do not profoundly constrain its occurrence should be identified for reforestation or agroforestry parklands using the studied species. Faidherbia albida is an important multipurpose and nitrogen-fixing tree, well known for its ecosystem services, and a keystone species for Evergreen Agriculture in Africa, with crop yields under its canopy often observed to double or triple (Hadgu et al., 2009). Agroforestry with F. albida significantly increases maize yields, demonstrating a direct link between this tree-based energy input and increased food security, especially given F. albida’s adaptation to drylands such as in the Sahel (Haskett et al., 2019). This was also found for maize production in Zambia (Yengwe et al., 2018a), where Faidherbia tree canopies showed to contribute to food security and mitigate the risk of crop failure on resource-poor smallholder farmers’ fields, especially in drought years. Similar results have been found using Faidherbia plant material as maize fertilizer in Nigeria (Chinke et al., 2022). Faidherbia trees increased soil mineral N, wheat water use efficiency and reduced heat stress, significantly increasing yield in the Central Rift Valley of Ethiopia (Desta et al., 2018; Sida et al., 2018b), not only in wheat but also in sorghum (Abdella et al., 2020). Furthermore, it has been shown that maize production and profitability could be maintained or improved through only partial pruning of F. albida in Ethiopia (Dilla et al., 2019a). In the latter, its use has been suggested as a mean to increase crop productivity, contain weed infestations, to produce livestock fodder, and as a source of nectar for honey production (Ereso, 2019), also having a considerable potential in carbon sequestration (Dilla et al., 2019b). Yengwe et al. (2018b) found that the improvement of soil fertility status by F. albida could be attributed to a combination of both long-term modifications of the soil biological and chemical properties, under the canopy, as well as short term litter fall addition. The global positive impact of F. albida on crop productivity and on soil enrichment has been recently revised by Sileshi et al. (2020).
Given the aridity of Cabo Verde islands and the possibility of contributing to the preservation and enrichment of the soil, which greatly aids in mitigating climate change, it is critical to reforest areas with a species well adapted to the present and future local climate. Furthermore, given the results obtained by Varela et al. (2022) regarding the climatic adaptation of one of the endemic trees to extreme aridity (Phoenix atlantica), the possibility of using consociations of both species should be considered in the future. Moreover, the possibility of using both species to improve the conditions for agricultural practices and for the recovery of other endemic and native species should also be investigated.
Finally, it is emphasized that knowledge of the geographical range of the native trees in Cabo Verde provides essential baseline data toward achieving the goals of the Paris Agreement, which are in the country’s agenda as a priority to conserve the fragile biodiversity of these islands. In this sense, we do hope that local authorities will benefit from this research and develop actions to promote sustainable reforestation, which should include native trees that are highly adapted to the local climate and could contribute to mitigate the effects of climate change.
5. Conclusion
Faidherbia albida occurs in Africa in forest galleries along rivers and is a frequent floristic element in open tropical deciduous forests and, mainly, savannas. In Cabo Verde, until the late 20th century, natural populations were significantly reduced due to its utility for livestock feeding and as firewood. Nowadays, this species is confined to temporary river zones, valleys or rocky areas, and only sporadically, as in Ribeira da Barca (Santiago Island), is it possible to find small patches of what the pristine savannas of F. albida in Cabo Verde might have been (Neto et al., 2020). Thus, the continental African savannas where most of the F. albida are found, currently have no correspondence in Cabo Verde, having been occupied by large-scale plantations of Prosopis juliflora or other non-native trees. Modelling the bioclimatic niche of F. albida in Cabo Verde, and constraining the potential distribution with LULC data, allowed to identify the environmental and anthropogenic constraints that might have affected F. albida distribution in the past, and restrain its future range, thereby enabling the establishment of priorities in its management. The knowledge of the geographical range of Cabo Verde native trees provides essential baseline data toward achieving the goals of the Paris Agreement, which are on the country’s agenda as a priority to conserve the fragile biodiversity of these islands. Thus, our methodological approach could be further extended to other native Cabo Verde trees in a straightforward manner. In this sense, we do hope that local authorities will benefit from this research, in the development of actions to promote reforestation, which should include native trees that are highly adapted to the local climate and could contribute to further mitigate the effects of climate change.
Data availability statement
The datasets presented in this study can be found in online repositories. The names of the repository/repositories and accession number(s) can be found in the article/Supplementary material.
Author contributions
All authors listed have made a substantial, direct, and intellectual contribution to the work and approved it for publication.
Funding
This research was funded by the Fundação para a Ciência e Tecnologia (FCT) and Aga Khan Development Network (AKDN) through the project CVAgrobiodiversity/333111699. DV was supported by FCT grant (SFRH/BD/135354/2017).
Acknowledgments
Special thanks to Silvia Catarino and Lara Dutra Silva for all their support in modelling. The authors thank the herbarium curator (Maria Cristina Duarte, FCUL) of the LISC Herbarium (IICT/ULisboa). We thank to Fundação para a Ciência e Tecnologia (FCT) and Aga Khan Development Network (AKDN) under the project CVAgrobiodiversity. Also to the research unit UID/AGR/04129/2020 (LEAF) and CIBIO-Açores funded by Portuguese National Funds through FCT, Portugal.
Conflict of interest
The authors declare no conflict of interest. The funders had no role in the design of the study; in the collection, analyses, or interpretation of data; in the writing of the manuscript, or in the decision to publish the results.
Publisher’s note
All claims expressed in this article are solely those of the authors and do not necessarily represent those of their affiliated organizations, or those of the publisher, the editors and the reviewers. Any product that may be evaluated in this article, or claim that may be made by its manufacturer, is not guaranteed or endorsed by the publisher.
Supplementary material
The Supplementary material for this article can be found online at: https://www.frontiersin.org/articles/10.3389/fevo.2023.1057852/full#supplementary-material
Footnotes
References
Abdella, M., Nigatu, L., and Akuma, A. (2020). Impact of parkland trees (Faidherbia albida Delile and Cordia africana Lam) on selected soil properties and sorghum yield in Eastern Oromia, Ethiopia. Agric. Forest. Fisheries 9, 54–73. doi: 10.11648/j.aff.20200903.13
Adjonou, K., Abotsi, K. E., Segla, K. N., Rabiou, H., Houetchegnon, T., Sourou, K. N. B., et al. (2020). Vulnerability of African Rosewood (Pterocarpus erinaceus, Fabaceae) natural stands to climate change and implications for silviculture in West Africa. Heliyon 6:e04031. doi: 10.1016/j.heliyon.2020.e04031
Allouche, O., Tsoar, A., and Kadmon, R. (2006). Assessing the accuracy of species distribution models: prevalence, kappa and the true skill statistic (TSS). J. Appl. Ecol. 43, 1223–1232. doi: 10.1111/j.1365-2664.2006.01214.x
Araújo, M., Pearson, R., Thuiller, W., and Erhard, M. (2005). Validation of species-climate impact models under climate change. Glob. Chang. Biol. 11, 1504–1513. doi: 10.1111/j.1365-2486.2005.001000.x
Arévalo, J. R., Delgado, J. D., Otto, R., Naranjo, A., Salas, M., and Fernández-Palacios, J. M. (2005). Distribution of alien vs. native plant species in roadside communities along an altitudinal gradient in Tenerife and Gran Canaria (Canary Islands). Perspect. Plant Ecol. Evol. Syst. 7, 185–202. doi: 10.1016/j.ppees.2005.09.003
Atanasso, J. A., Salako, K. V., Mensah, S., Tohoun, R. J., Djossa, B. A., Kakaï, R. G., et al. (2021). Spatial distribution patterns of Afzelia africana (Fabaceae – Detarioideae) in a tropical savanna of Benin: implications for management. Plant Ecol. Evol. 154, 362–375. doi: 10.5091/PLECEVO.2021.1713
Barbet-Massin, M., Jiguet, F., Albert, C. H., and Thuiller, W. (2012). Selecting pseudo-absences for species distribution models: How, where and how many? Methods Ecol. Evol. 3, 327–338. doi: 10.1111/j.2041-210X.2011.00172.x
Barnes, R., and Fagg, C. (2003). Faidherbia albida monograph and annotated bibliography. Oxford: Oxford Tropical Forestry Papers, 289.
Booth, T. H. (2018). Species distribution modelling tools and databases to assist managing forests under climate change. For. Ecol. Manag. 430, 196–203. doi: 10.1016/j.foreco.2018.08.019
Boyce, M. S., Vernier, P. R., Nielsen, S. E., and Schmiegelow, F. K. A. (2002). Evaluating resource selection functions mark. Ecol. Model. 157, 281–300. doi: 10.1016/S0304-3800(02)00200-4
Casajus, N., Perie, C., Casajus, N., Périé, C., Logan, T., Lambert, M., et al. (2016). An objective approach to select climate scenarios when projecting species distribution under climate change an objective approach to select climate scenarios when projecting species distribution under climate change. PLoS One 11:e0152495. doi: 10.5061/dryad.1sf74
Castilla-Beltrán, A., de Nascimento, L., Fernández-Palacios, J. M., Fonville, T., Whittaker, R. J., Edwards, M., et al. (2019). Late Holocene environmental change and the anthropization of the highlands of Santo Antão Island, Cabo Verde. Palaeogeogr. Palaeoclimatol. Palaeoecol. 524, 101–117. doi: 10.1016/j.palaeo.2019.03.033
Castilla-Beltran, A., de Nascimento, L., Fernandez-Palacios, J. M., Whittaker, R. J., Romeiras, M. M., Cundy, A. B., et al. (2021). Effects of Holocene climate change, volcanism and mass migration on the ecosystem of a small, dry island (Brava, Cabo Verde). J. Biogeogr. 48, 1392–1405. doi: 10.1111/jbi.14084
Catarino, S., Romeiras, M. M., Pereira, J. M., and Figueira, R. (2021). Assessing the conservation of Miombo timber species through an integrated index of anthropogenic and climatic threats. Ecol. Evol. 11, 9332–9348. doi: 10.1002/ece3.7717
CCKP (2019). World Bank climate change knowledge portal | for global climate data and information! Available at: https://climateknowledgeportal.worldbank.org/country/cape-verde (Accessed August 1, 2020).
Chang, X.-Y., Chen, B.-M., Liu, G., Zhou, T., Jia, X.-R., and Peng, S.-L. (2015). Effects of climate change on plant population growth rate and community composition change. PLoS One 10:e0126228. doi: 10.1371/journal.pone.0126228
Chaturvedi, R. K., Joshi, J., Jayaraman, M., Bala, G., and Ravindranath, N. H. (2012). Multi-model climate change projections for India under representative concentration pathways. Curr. Sci. 103, 791–802. doi: 10.2307/24088836
Chen, X., and Leites, L. (2020). The importance of land-use legacies for modeling present-day species distributions. Landsc. Ecol. 35, 2759–2775. doi: 10.1007/S10980-020-01119-0
Chinke, N. M., Hamzat, S., Oyinlola, E. Y., Idris, S., and Yusif, S. A. (2022). Assessment of nitrogen mineralization of Faidherbia albida and its effect on maize (Zea mays L.) growth on an alfisol in Samaru, Northern Guinea Savanna of Nigeria. J. Agric. Environ. 18, 167–181.
Cienciala, E., Centeio, A., and Blazek, P., Cruz Gomes Soares, M. da, & Russ, R. (2013). Estimation of stem and tree level biomass models for Prosopis juliflora pallida applicable to multi-stemmed tree species. Trees - Struct. Funct., 27, 1061–1070. doi: 10.1007/s00468-013-0857-1
CILSS (2016). Landscapes of West Africa—A window on a changing world. Comité Permanent Inter-états de Lutte contre la Sécheresse dans le Sahel.
Costa, H., Medeiros, V., Azevedo, E. B., and Silva, L. (2013). Evaluating ecological-niche factor analysis as a modelling tool for environmental weed management in island systems. Weed Res. 53, 221–230. doi: 10.1111/wre.12017
Deblauwe, V., Droissart, V., Bose, R., Sonké, B., Blach-Overgaard, A., Svenning, J. C., et al. (2016). Remotely sensed temperature and precipitation data improve species distribution modelling in the tropics. Glob. Ecol. Biogeogr. 25, 443–454. doi: 10.1111/geb.12426
Desta, K. N., Lisanenwork, N., and Muktar, M. (2018). Physico-chemical properties of soil under the canopies of Faidherbia albida (Delile) A. Chev and Acacia tortilis (Forssk.) Hayen in park land agroforestry system in Central Rift Valley, Ethiopia. J. Hortic. Forest. 10, 1–8. doi: 10.5897/JHF2016.0491
Dilla, A. M., Smethurst, P. J., Barry, K., and Parsons, D. (2019b). Preliminary estimate of carbon sequestration potential of Faidherbia albida (Delile) A. Chev in an agroforestry parkland in the Central Rift Valley of Ethiopia. Forest. Trees Livelihoods 28, 79–89. doi: 10.1080/14728028.2018.1564146
Dilla, A. M., Smethurst, P. J., Barry, K., Parsons, D., and Denboba, M. A. (2019a). Tree pruning, zone and fertiliser interactions determine maize productivity in the Faidherbia albida (Delile) A. Chev parkland agroforestry system of Ethiopia. Agrofor. Syst. 93, 1897–1907. doi: 10.1007/s10457-018-0304-9
Dobson, A. J., and Barnett, A. G. (2018). An introduction to generalized linear models. 4th Edn. New York: Chapman and Hall/CRC.
Duarte, M. C., Gomes, I., Catarino, S., Brilhante, M., Gomes, S., Rendall, A., et al. (2022). Diversity of useful plants in Cabo Verde Islands: a biogeographic and conservation perspective. Plan. Theory 11:1313. doi: 10.3390/plants11101313
Duarte, M. C., Rego, F., Romeiras, M. M., and Moreira, I. (2008). Plant species richness in the Cape Verde Islands eco-geographical determinants. Biodivers. Conserv. 17, 453–466. doi: 10.1007/s10531-007-9226-y
Elith, J., H. Graham, C., P. Anderson, R., Dudík, M., Ferrier, S., Guisan, A., et al. (2006). Novel methods improve prediction of species’ distributions from occurrence data. Ecography 29, 129–151. doi: 10.1111/j.2006.0906-7590.04596.x
Emptage, M., and Dobson, A. J. (2008). An Introduction to generalized linear models. Florida: Chapman & Hall/CRC.
Ereso, T. (2019). The role of Faidherbia albida tree species in parkland agroforestry and its management in Ethiopia. J. Hortic. Forest. 11, 42–47. doi: 10.5897/JHF2018.0570
Fahrig, L. (2003). Effects of habitat fragmentation on biodiversity. Annu. Rev. Ecol. Evol. Syst. 34, 487–515. doi: 10.1146/annurev.ecolsys.34.011802.132419
Feeley, K. J., Bravo-Avila, C., Fadrique, B., Perez, T. M., and Zuleta, D. (2020). Climate-driven changes in the composition of New World plant communities. Nat. Clim. Chang. 10, 965–970. doi: 10.1038/s41558-020-0873-2
Ferrarini, A., Dai, J., Bai, Y., and Alatalo, J. M. (2019). Redefining the climate niche of plant species: A novel approach for realistic predictions of species distribution under climate change. Sci. Total Environ. 671, 1086–1093. doi: 10.1016/j.scitotenv.2019.03.353
Fick, S., and Hijmans, R. (2017). Worldclim 2: new 1-km spatial resolution climate surfaces for global land areas. Int. J. Climatol. 37, 4302–4315. doi: 10.1002/joc.5086
Florencio, M., Patiño, J., Nogué, S., Traveset, A., Borges, P. A. V., Schaefer, H., et al. (2021). Macaronesia as a fruitful arena for ecology, evolution, and conservation biology. Front. Ecol. Evol. 9:718169. doi: 10.3389/fevo.2021.718169
García-Valdés, R., Svenning, J. C., Zavala, M. A., Purves, D. W., and Araújo, M. B. (2015). Evaluating the combined effects of climate and land-use change on tree species distributions. J. Appl. Ecol. 52, 902–912. doi: 10.1111/1365-2664.12453
Gorel, A. P., Duminil, J., Doucet, J. L., and Fayolle, A. (2019). Ecological niche divergence associated with species and populations differentiation in Erythrophleum (Fabaceae, Caesalpinioideae). Plant Ecol. Evol. 152, 41–52. doi: 10.5091/plecevo.2019.1543
Guisan, A., Edwards, T. C., and Hastie, T. (2002). Generalized linear and generalized additive models in studies of species distributions: setting the scene. Ecol. Model. 157, 89–100. doi: 10.1016/S0304-3800(02)00204-1
Hadgu, K. M., Kooistra, L., Rossing, W. A. H., and van Bruggen, A. H. C. (2009). Assessing the effect of Faidherbia albida based land use systems on barley yield at field and regional scale in the highlands of Tigray, Northern Ethiopia. Food Secur. 1, 337–350. doi: 10.1007/s12571-009-0030-2
Haskett, J. D., Simane, B., and Smith, C. (2019). Energy and climate change mitigation benefits of Faidherbia albida agroforestry in Ethiopia. Front. Environ. Sci. 7, 1–15. doi: 10.3389/fenvs.2019.00146
Hegel, T. M., Cushman, S. A., Evans, J., and Huettmann, F. (2010). “Current state of the art for statistical modelling of species distributions” in Spatial complexity, informatics, and wildlife conservation (Japan: Springer), 273–311.
Hijmans, R. J., Etten, J.van, Sumner, M., Cheng, J., and Baston, D. (2020). CRAN - Package raster (3.4-5). Available at: https://cran.r-project.org/web/packages/raster/index.html (Accessed December 24, 2020).
IPCC (2018a). Global warming of 1.5°C an IPCC special report on the impacts of global warming of 1.5°C above pre-industrial levels and related global greenhouse gas emission pathways, in the context of strengthening the global response to the threat of climate change, sustainable development, and efforts to eradicate poverty headline statements from the summary for policymakers* understanding global warming of 1.5°C. Available at: https://www.ipcc.ch/site/assets/uploads/sites/2/2018/07/sr15_headline_statements.pdf
IPCC (2018b). Headline statements from the summary for policymakers. Global warming of 1.5°C. an IPCC special report on the impacts of global warming of 1.5°C above pre-industrial levels and related global greenhouse gas emission pathways, in the context of strengthening the global response to the threat of climate change, 1–2.
IPCC (2022). Climate change 2022: Impacts, adaptation, and vulnerability. Switzerland: Cambridge University Press.
Lindskog, P., and Delaite, B. (1996). Degrading land: an environmental history perspective of the Cape Verde Islands. Environ. Hist. 2, 271–290. doi: 10.3197/096734096779522266
Lu, T., Brandt, M., Tong, X., Hiernaux, P., Leroux, L., Ndao, B., et al. (2022). Mapping the abundance of Faidherbia albida trees in Senegal with Sentinel-2 time series data. Remote Sensing, Submitted.
Luckow, M., Miller, J. T., Murphy, D. J., and Livshultz, T. (2003). “A phylogenetic analysis of the Mimosoideae (Leguminosae) based on chloroplast DNA sequence data” in Advances in legume systematics, part 10, higher level systematics. eds. B. B. Klitgaard and A. Bruneau (Kew, UK: Royal Botanic Gardens), 197–220.
Marmion, M., Parviainen, M., Luoto, M., Heikkinen, R. K., and Thuiller, W. (2009). Evaluation of consensus methods in predictive species distribution modelling. Divers. Distrib. 15, 59–69. doi: 10.1111/j.1472-4642.2008.00491.x
Monteiro, F., Fortes, A., Ferreira, V., Essoh, A.P., Gomes, I., and Correia, M., A., & Romeiras, M.M., (2020). Status and trends in Cabo Verde agriculture. Agronomy, 10:74. doi: 10.3390/agronomy10010074
Ndao, B., Leroux, L., Hema, A., Diouf, A. A., Bégué, A., and Sambou, B. (2022). Tree species diversity analysis using species distribution models: A Faidherbia albida parkland case study in Senegal. Ecol. Indic. 144:109443. doi: 10.1016/j.ecolind.2022.109443
Neto, C., Costa, J. C., Figueiredo, A., Capelo, J., Gomes, I., Vitoria, S., et al. (2020). The role of climate and topography in shaping the diversity of plant communities in Cabo Verde Islands. Diversity 12:80. doi: 10.3390/d12020080
Newman, D., Nimon, K., Walker, D. A., Lieberman, M. G., and Morris Florida, J. D. (2010). Comparing OLS and HLM models and the questions they answer: potential concerns for type VI errors. Multiple Linear Reg. Viewpoints 36:33.
Norder, S. J., Lima, R. F., De Nascimento, L., Lim, J. Y., Fernandez-Palacios, J. M., Romeiras, M. M., et al. (2020). Global change in microcosms: environmental and societal predictors of land cover change on the Atlantic Ocean Islands. Anthropocene 30:100242. doi: 10.1016/j.ancene.2020.100242
Noulèkoun, F., Chude, S., Zenebe, A., and Birhane, E. (2017). Climate change impacts on Faidherbia albida (Delile) A. Chev. Distribution in dry lands of Ethiopia. Afr. J. Ecol. 55, 233–243. doi: 10.1111/aje.12345
Nyairo, R., and Machimura, T. (2020). Potential effects of climate and human influence changes on range and diversity of nine Fabaceae species and implications for nature’s contribution to people in Kenya. Climate 8, 1–19. doi: 10.3390/cli8100109
Olehowski, C., Naumann, S., Fischer, D., and Siegmund, A. (2008). Geo-ecological spatial pattern analysis of the island of Fogo (Cape Verde). Glob. Planet. Chang. 64, 188–197. doi: 10.1016/j.gloplacha.2008.09.006
Parmesan, C., and Hanley, M. E. (2015). Plants and climate change: complexities and surprises. Ann. Bot. 116, 849–864. doi: 10.1093/aob/mcv169
Pearce, J. L., and Boyce, M. S. (2006). Modelling distribution and abundance with presence-only data. J. Appl. Ecol. 43, 405–412. doi: 10.1111/j.1365-2664.2005.01112.x
Perrin, G., Rapinel, S., Hubert-Moy, L., and Bioret, F. (2020). Bioclimatic dataset of metropolitan France under current conditions derived from the WorldClim model. Data Brief 31:105815. doi: 10.1016/j.dib.2020.105815
POWO - Plants of the World Online (2021). Available at: http://powo.science.kew.org [Accessed September 15, 2021].
Prasad, A. M., Iverson, L. R., and Liaw, A. (2006). Newer classification and regression tree techniques: bagging and random forests for ecological prediction. Ecosystems 9, 181–199. doi: 10.1007/s10021-005-0054-1
QGIS Development Team (2021). QGIS geographic information system. Open Source Geospatial Foundation Project. Available at: http://qgis.osgeo.org [Accessed April 17, 2021].
R Development Core Team (2020). R: A language and environment for statistical computing. R Foundation for Statistical Computing. Available at: http://www.r-project.org/index.html (Accessed December 26, 2020).
Ren-Yan, D., Xiao-Quan, K., Min-Yi, H., Wei-Yi, F., and Zhi-Gao, W. (2014). The predictive performance and stability of six species distribution models. PLoS One 9:e112764. doi: 10.1371/journal.pone.0112764
Rigby, R. A., Stasinopoulos, D. M., and Lane, P. W. (2005). Generalized additive models for location, scale and shape. Journal of the Royal Statistical Society. Series C. Appl. Stat. 54, 507–554. doi: 10.1111/j.1467-9876.2005.00510.x
Rivas-Martínez, S., Lousã, M., Costa, J. C., and Duarte, M. C. (2017). Geobotanical survey of Cabo Verde Islands (West Africa). Int. J. Geobot. Res. 7, 1–103.
Romeiras, M. M., Carine, M., Duarte, M. C., Catarino, S., Dias, F. S., and Borda-de-Água, L. (2020). Bayesian methods to analyze historical collections in time and space: a case study using Cabo Verde Endemic Flora. Front. Plant Sci. 11:278. doi: 10.3389/fpls.2020.00278
Romeiras, M. M., Catarino, S., Gomes, I., Fernandes, C., Costa, J. C., Caujapé-Castells, J., et al. (2016). IUCN red list assessment of the Cape Verde endemic flora: towards a global strategy for plant conservation in Macaronesia. Bot. J. Linn. Soc. 180, 413–425. doi: 10.1111/boj.12370
Romeiras, M. M., Duarte, M. C., Santos-Guerra, A., Carine, M. A., and Francisco-Ortega, J. (2014). Botanical exploration of the Cape Verde islands: from the pre-Linnaean records and collections to the late 18th century accounts and expeditions. Taxon 63, 625–640. doi: 10.12705/633.37
Romeiras, M. M., Pena, A. R., Menezes, T., Vasconcelos, R., Monteiro, F., Paulo, O. S., et al. (2019). Shortcomings of phylogenetic studies on recent radiated insular groups: A meta-analysis using Cabo Verde biodiversity. Int. J. Mol. Sci. 20:2782. doi: 10.3390/ijms20112782
Santini, L., Benítez-López, A., Maiorano, L., Čengić, M., and Huijbregts, M. A. J. (2021). Assessing the reliability of species distribution projections in climate change research. Divers. Distrib. 27, 1035–1050. doi: 10.1111/ddi.13252
Sida, T. S., Baudron, F., Deme, D. A., Tolera, M., and Giller, K. E. (2018a). Excessive pruning and limited regeneration: are Faidherbia albida parklands heading for extinction in the central Rift Valley of Ethiopia? Land Degrad. Dev. 29, 1623–1633. doi: 10.1002/ldr.2959
Sida, T. S., Baudron, F., Kim, H., and Giller, K. E. (2018b). Climate-smart agroforestry: Faidherbia albida trees buffer wheat against climatic extremes in the Central Rift Valley of Ethiopia. Agric. For. Meteorol. 248, 339–347. doi: 10.1016/j.agrformet.2017.10.013
Sileshi, G. W., Teketay, D., Gebrekirstos, A., and Hadgu, K. (2020). “Sustainability of Faidherbia albida-based agroforestry in crop production and maintaining soil health” in Agroforestry for degraded landscapes: Recent advances and emerging challenges. eds. J. C. Dagar, S. R. Gupta, and D. Teketay, vol. 2 (Singapore: Springer), 349–369.
Silva, L., Bento Elias, R., and Silva, L. (2021). Modelling invasive alien plant distribution: A literature review of concepts and bibliometric analysis. Environ. Model. Softw. 145:105203. doi: 10.1016/J.ENVSOFT.2021.105203
Silva, L., Costa, H., de Azevedo, E. B., Medeiros, V., Alves, M., Elias, R. B., et al. (2017). Modelling native and invasive woody species: A comparison of ENFA and MaxEnt applied to the Azorean forest. Springer Proc. Math. Stat. 195, 415–444. doi: 10.1007/978-3-319-55236-1_20
Silva, L., de Azevedo, E. B., Reis, F. V., Elias, R. B., and Silva, L. (2019). Limitations of species distribution models based on available climate change data: A case study in the Azorean forest. Forests 10, 1–29. doi: 10.3390/f10070575
Singer, A., Millat, G., Staneva, J., and Kröncke, I. (2017). Modelling benthic macrofauna and seagrass distribution patterns in a North Sea tidal basin in response to 2050 climatic and environmental scenarios. Estuar. Coast. Shelf Sci. 188, 99–108. doi: 10.1016/j.ecss.2017.02.003
Sylla, M. B., Nikiema, P. M., Gibba, P., Kebe, I., and Klutse, N. A. B. (2016). “Climate change over West Africa: recent trends and future projections” in Adaptation to climate change and variability in rural West Africa. eds. J. A. Yaro and J. Hesselberg (Springer International Publishing), 25–40.
Symon, C. (2013). Climate change: action, trends and implications for business: the IPCC’s fifth assessment report, working group 1. Available at: http://www.europeanclimate.org/documents/IPCCWebGuide.pdf
Thuiller, W. (2003). BIOMOD - optimizing predictions of species distributions and projecting potential future shifts under global change. Glob. Chang. Biol. 9, 1353–1362. doi: 10.1046/j.1365-2486.2003.00666.x
Thuiller, W., Georges, D., Engler, R., and Breiner, F. (2016). Ensemble platform for species distribution modelling (3.3-7.1). Available at: https://r-forge.r-project.org/R/?group_id=302 (Accessed June 18, 2020).
Thuiller, W., Lavergne, S., Roquet, C., Boulangeat, I., Lafourcade, B., and Araujo, M. B. (2011). Consequences of climate change on the tree of life in Europe. Nature 470, 531–534. doi: 10.1038/nature09705
USGS (2022). Land use and land cover trends in West Africa | West Africa. Landscapes of West Africa: A Window on a Changing World. Available at: https://eros.usgs.gov/westafrica/land-cover/land-use-and-land-cover-trends-west-africa (Accessed February 28, 2022).
Vandenbeldt, R. J. (1992). “Faidherbia albida in the west African semi-arid tropics.” in proceedings of a workshop, 22-26 Apr 1991, Niamey, Niger. (in En. Summaries in En, Fr, Es.) Patancheru, A.P. 502 324, India: International Crops Research Institute for the Semi-Arid Tropics; and Nairobi, Kenya: International Centre for Research in Agrofor. International Centre for Research in Agroforestry.
Varela, D., Monteiro, F., Vidigal, P., Silva, L., and Romeiras, M. M. (2020). Mechanisms implemented for the sustainable development of agriculture: an overview of Cabo Verde performance. Sustainability 12, 3–4. doi: 10.3390/su12145855
Varela, D., Romeiras, M. M., and Silva, L. (2022). Implications of climate change on the distribution and conservation of Cabo Verde endemic trees. Glob. Ecol. Conser. 34:e02025. doi: 10.1016/j.gecco.2022.e02025
Varela-Lopes, G. E., and Molion, L. C. B. (2014). Precipitation patterns in Cape Verde Islands: Santiago Island Case study. Atmos. Climate Sci. 4, 854–865. doi: 10.4236/acs.2014.45075
Wang, J., Wang, Y., Feng, J., Chen, C., Chen, J., Long, T., et al. (2019). Differential responses to climate and land-use changes in threatened Chinese Taxus species. Forests 10:16. doi: 10.3390/f10090766
Wooldridge, J. M. (2008). Introductory econometrics: A modern approach. 4th Edn. Mason: Thomson Southwestern Ohio.
Yengwe, J., Amalia, O., Lungu, O. I., and De Neve, S. (2018a). Quantifying nutrient deposition and yield levels of maize (Zea mays) under Faidherbia albida agroforestry system in Zambia. Eur. J. Agron. 99, 148–155. doi: 10.1016/j.eja.2018.07.004
Yengwe, J., Gebremikael, M. T., Buchan, D., Lungu, O., and De Neve, S. (2018b). Effects of Faidherbia albida canopy and leaf litter on soil microbial communities and nitrogen mineralization in selected Zambian soils. Agrofor. Syst. 92, 349–363. doi: 10.1007/s10457-016-0063-4
Keywords: tropical dry islands, native tree species, species distribution models, climate change, habitat suitability, Cabo Verde, West Africa
Citation: Varela D, Romeiras MM and Silva L (2023) Present and future distribution of Faidherbia albida in Cabo Verde as revealed by climatic modelling and LULC analysis. Front. Ecol. Evol. 11:1057852. doi: 10.3389/fevo.2023.1057852
Edited by:
Jiang Jiang, Nanjing Forestry University, ChinaReviewed by:
Lina Cui, Nanjing Forestry University, ChinaBabacar Ndao, Centre de Suivi Ecologique, Senegal
Copyright © 2023 Varela, Romeiras and Silva. This is an open-access article distributed under the terms of the Creative Commons Attribution License (CC BY). The use, distribution or reproduction in other forums is permitted, provided the original author(s) and the copyright owner(s) are credited and that the original publication in this journal is cited, in accordance with accepted academic practice. No use, distribution or reproduction is permitted which does not comply with these terms.
*Correspondence: Maria M. Romeiras, mmromeiras@isa.ulisboa.pt