Non-viviparous pre-dispersal seed germination in Amaranthaceae in the cold deserts of Central Asia
- 1College of Life Sciences, Xinjiang Agricultural University, Urümqi, China
- 2Key Laboratory of Ministry of Education of Grassland Resources and Ecology in Western Arid Region, College of Grassland Science, Xinjiang Agricultural University, Urümqi, China
- 3Xinjiang Key Laboratory of Grassland Resources and Ecology, College of Grassland Science, Xinjiang Agricultural University, Urümqi, China
- 4Department of Biology, University of Kentucky, Lexington, KY, United States
- 5Department of Plant and Soil Sciences, University of Kentucky, Lexington, KY, United States
In the broad context of understanding the relationship between timing of seed germination and adaptation of a plant species to its habitat, the purpose of this study was to purse an observation of pre-dispersal seed germination of Salsola brachiata (Amaranthaceae) in late winter 2021 in the Amaranthaceae species-rich cold deserts in northwest China (Central Asia). We searched for pre-dispersal germination in species of Amaranthaceae growing in sand dunes (S), salt deserts (SD) and gravel deserts (GD). We examined 69 species in 155 populations in autumn 2021 and 52 species in 12 populations in early spring 2022. No seeds of any of the 69 species germinated on the mother plants in autumn 2021, while 30 of 52 species (57.7%) did so during snowmelt in early spring 2022. The rank order of species with few to many seeds germinated on the mother plants was annuals (66.7%) > small shrubs (23.3%) > small trees (6.7%) > shrubs (3.3%). The number of species in S, SD, and GD with pre-dispersal germinated seeds was 16 of 27 (59.3%), 15 of 31 (48.4%), and 15 of 30 (50.0%), respectively. The high species occurrence of pre-dispersal germination in early spring suggested that it might be adaptive in the unpredictable-rainfall growing-season environment of the cold deserts of Central Asia, a center of diversity of Amaranthaceae. However, preliminary studies on seedling/juvenile survival of S. brachiata showed that those from post-dispersal soil-germinated seeds had the best survival, suggesting that pre-dispersal seed germination may be maladaptive.
Introduction
Timing of seed germination plays a key role in the adaptation of plant species to their habitat, in particular because it affects the expression of life history traits and fitness components across the plant life cycle, e.g., seedling survival and seed production (Donohue et al., 2010; Postma and Ågren, 2022). The time of germination involves not only dormancy-break of the seeds but also their germination requirements after dormancy is broken. Thus, although dormancy may be broken germination will not occur unless the germination requirements of the seeds overlap with the environmental conditions in the habitat. Seed ecologists are interested in the requirements for both dormancy break and germination, and much attention has been given to the actual time of germination in the field because this provides insight into the relationship between the species and its habitat (Baskin and Baskin, 1972; Lu et al., 2014a,b, 2017, 2020; Maleki et al., 2022).
In late February 2021, the first author observed that some seeds attached to dead stems of Salsola brachiata (Amaranthaceae) growing in a cold desert of northern Xinjiang Province China (Central Asia) had germinated. At the time pre-dispersal seed germination was observed, the dead stems and seeds of this annual plant were very moist due to melting of the snow lodged on them. Subsequently, pre-dispersal seed germination was observed for other members of the Amaranthaceae growing in the cold desert, i.e., Anabasis elatior, Atriplex micrantha, and Kochia prostrata. These observations of pre-dispersal germination raised three questions. (1) How common is pre-dispersal germination among species of Amranthaceae in the cold deserts of northwest China? (2) Does pre-dispersal germination occur only in late winter/early spring during the time of snowmelt? (3) What is the consequence of pre-dispersal germination on seedling/juvenile survival?
To address the first two questions, a field survey of numerous species of Amaranthaceae growing in these cold deserts was conducted in autumn 2021 and spring 2022. To address the question related to seedling survival, we monitored the survival of S. brachiata seedlings/juveniles resulting from pre-dispersal and post-dispersal (sown on soil) germination in spring 2022. We predicted that pre-dispersal germination (1) is limited to the snowmelt period of late winter/early spring when moisture in the habitat is high, and (2) many of the pre-dispersal seedlings fail to become established in the soil; that is, pre-dispersal seed germination is maladaptive.
The cold deserts in northern Xinjiang Province of China (Central Asia) are the main distribution area of Amaranthaceae species in the world (Mao, 1994). In this area, Amaranthaceae species include very common annual plants, semi-shrubs, and shrubs, but perennials and small trees are present. Also, species of Amaranthaceae are dominant or otherwise present in a diversity of habitat types (e.g., sand dune, salt desert, and gravel desert) [Xinjiang General Exploration Team of Chinese Academy of Sciences (CAS), and Institute of Botany of CAS, 1978]. Thus, we determined the occurrence of pre-dispersal seed germination of Amaranthaceae species in sand dunes (S), gravel deserts (GD) and salt deserts (SD) in the cold deserts of northern Xinjiang at seed maturity in autumn and at the onset of the growing season (snowmelt) in late winter/early spring.
Materials and methods
The study area
The cold deserts in northern Xinjiang have a typical continental arid/semiarid climate with a low amount of precipitation and high evaporation (Lioubimtseva and Cole, 2006). Depending on location, mean monthly annual temperature ranges from 4.0°C to 9.0°C, and the range of mean temperatures of the coldest (January) and hottest (July) months is −32.8°C to −15.0°C and 25.0°C–40.5°C, respectively. Average annual precipitation (including rain and snow) is 160–300 mm, about two-thirds of which falls in spring and summer, and the snow that falls in winter begins to melt in March or April (Huang et al., 2017). Annual potential evaporation is 1,500–3,000 mm (Zhang et al., 2016). Additionally, rainfall is highly variable among seasons and years, but generally rainfall is higher in spring than in autumn (Su et al., 2007; Shi and Sun, 2008). Further, water from snowmelt increases soil water availability in spring (Bie et al., 2016).
Vegetation cover is about 30%, and the dominant plants are small trees (Haloxylon species), shrubs (Calligonum, Caragana, Ephedra, Nitraria, Reaumuria, and Tamarix species), semi-shrubs, and dwarf semi-shrubs (Anabasis and Artemisia species), but many annuals (e.g., Agriophyllum and Salsola species) and herbaceous perennials (e.g., Allium and Astragalus species) are present (Zhang et al., 2016; Li et al., 2020). The proportion of vegetation cover, especially for herbaceous annuals and herbaceous perennials, varies with precipitation, being strongly associated with inter-annual and intra-annual precipitation patterns (Zhang et al., 2016; Fang et al., 2019; Li et al., 2020). The cold deserts of northern Xinjiang, like those in other regions, have salt lakes and salt pans with associated halophytic vegetation (Soriano, 1983; Walter and Box, 1983; West, 1983).
Survey of species with pre-dispersal seed germination
Species of Amaranthaceae are very common in the cold deserts in northern Xinjiang. Flowering occurs from early April to mid-September (Commissione Redactorum Florae Xinjiangensis, 1994), and fruits mature in late summer-early autumn [Xinjiang General Exploration Team of Chinese Academy of Sciences (CAS), and Institute of Botany of CAS, 1978]. Although some seeds are dispersed in autumn, many nondispersed seeds become covered by snow and are not dispersed until spring. The mature fruit (utricle) with two permanently attached bracteoles is the dispersal/germination unit, and in the field they germinate in late winter/early spring.
The objective of this part of the study was to survey the three kinds of typical habitats (i.e., S, GD, and SD) of Amaranthaceae species in the cold deserts of northern Xinjiang for pre-dispersal germination in autumn and spring. Thirty plants for each species were chosen haphazardly from each kind of habitat to observe pre-dispersal seed germination. If a plant of a species was observed with germinating seeds, the species was considered to have pre-dispersal seed germination. All species observed with pre-dispersal germination had few to many germinating seeds on the plants; especially the annual species had many germinating seeds. Only a few species, e.g., the trees Haloxylon persicum and Haloxylon ammodendron, had a relatively low number of seeds germinating on the plants, probably due to low snow cover on the branches and thus a low moisture level for the seeds. The number of species of Amaranthaceae with pre-dispersal seed germination was determined in each of the three kinds of habitats. Based on the Xinjiang General Exploration Team of Chinese Academy of Sciences (CAS), and Institute of Botany of CAS (1978), habitat types, life forms [i.e. annual herb (AH), shrub (S), semi-shrub (SS), or small tree (ST)] were recorded. For each species, pre-dispersal seed germination frequency (GF) was calculated as GF = GS/(GS + NGS), where GS and NGS are the number of sites with and without pre-dispersal seed germination, respectively. Information on geographical locations (i.e., longitude, latitude, and altitude) at the sampling sites was obtained using a GARMIN eTrex20 Global Positioning System (Jiaming Avionics Enterprise Management Co., Ltd., Shanghai, China).
Autumn
Amaranthaceae species were observed for pre-dispersal germinated seeds in a total of 155 sites (i.e., 34, 62, and 59 for S, GD, and SD, respectively; Figure 1) between fruit ripening and before snowfall in late September to early November 2021.
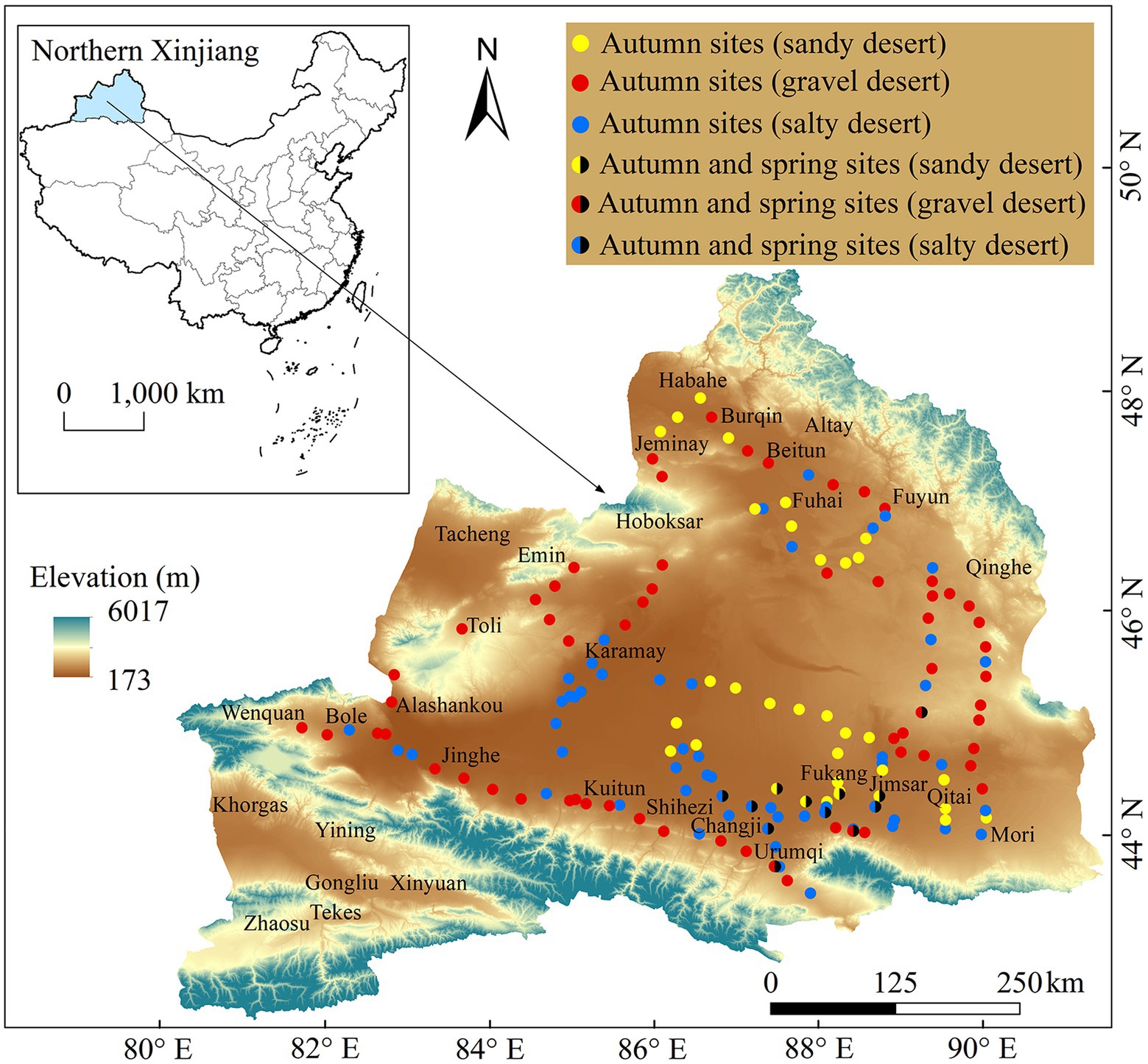
Figure 1. Sampling sites for pre-dispersal seed germination in Amaranthaceae species in the three habitat types in cold deserts of northern Xinjiang, China, for autumn 2021 and spring 2022.
Spring
In the cold deserts of northern Xinjiang, temperatures increase quickly in early spring, and the snowmelt period is short. Seeds on mother plants can germinate during the short period of snow melting in late winter/early spring. Twelve sites (i.e., four, three, and five of S, GD, and SD, respectively Figure 1) were evaluated during the short snowmelt period from early March to early April 2022. For each species, type of habitat and life form were recorded, and GF was calculated as described above.
Growth and development stages of seedlings
Growth and development stages of seedlings from pre-dispersal seed germination were monitored for each species until seedling detachment from their mother plant and establishment in the soil.
Survival and growth of Salsola brachiata seedlings/juveniles
The objective of this part of the study was to do a preliminary evaluation of seedling/juvenile survival in an experimental garden. On 1 November 2021, 100 dead plants of Salsola brachiata with mature fruits were collected from a natural population on Yamalic Hill, a suburb of Urümqi city near the southern edge of the cold deserts of northern Xinjiang, China (43°48′ N, 87°34′ E, 966 m a.s.l.). These plants were transplanted to bare soil in a 0.5 m × 1.0 m plot in the experimental garden located on the campus of Xinjiang Agricultural University, Urümqi, China, about 3 km from the population site. During snowmelt in early March 2022, seedlings from (1) pre-dispersal germinated seeds (S1), (2) soil-germinated seeds that had dispersed in autumn and thus under snow all winter (S2), and (3) soil-germinated seeds that were dispersed during snowmelt in spring 2022 (S3) were monitored for survival.
The S1 seedlings were from seeds that germinated on the transplanted dead mother plants and then after about 2–3 days had fallen to the soil. Ten fallen S1 seedlings were transplanted into each of three subplots of a 0.3 m × 0.5 m plot.
On Yamalic Hill, we observed that some seeds of S. brachiata were naturally dispersed during autumn (before snowfall) and in winter and the next spring. To ensure that there were seeds on the soil in the garden, we sowed seeds on the soil and on the snow surface. For S2 seedlings, 1,000 seeds were sown on soil in a 0.3 m × 0.5 m plot on 1 November 2021. When S2 seedlings emerged in spring, the plot was divided three smaller plots to monitor survival; there were 20, 15, and 15 seedlings in each of the three smaller plots. For S3 seedlings, 1,000 seeds were sown on the snow surface in a 0.3 m × 0.5 m plot on 24 February 2022. After S3 seedlings emerged, the plot was divided three smaller plots to monitor survival; there were 20, 15, and 15 seedlings in each of the three smaller plots. Thus, there was one plot each for S1, S2, and S3 seedlings/juveniles, and each plot was divided into three 0.16 × 0.10 m plots.
The S1 seedlings were monitored from the time they fell to the soil (6 March 2022) until they reached the four-leaf stage (6 April 2022). Since the S2 seedlings were covered by deep snow, and we could not determine the time of germination; we only saw them on 6 March after the snow had melted. Seeds for the S3 seedlings that were sown on the snow surface did not germinate until all the snow had melted (6 March), and then the seeds were on the soil surface. S2 and S3 seedlings were monitored from the time of germination (3 March 2022) until they reached the four-leaf stage (6 April 2022). In the experimental garden, seedlings were subjected to natural temperature and soil moisture conditions.
Another set of S1, S2, and S3 seedlings was marked with toothpicks to which different colors of thread were tied. After 0 (day of marking), 3, 6, 11, 16, 21, and 27 days of growth, five S1, S2, and S3 seedlings each were harvested and their roots washed free of soil. Then, the seedlings were oven-dried at 80°C for 48 h, and the dry mass of each seedling was determined by weighing using an electronic-balance (0.0001 g). The four-leaf stage at 27 days of growth was considered to be the end of the seedling establishment stage.
Data analysis
Correlative analyses were used to determine the relationship between pre-dispersal seed germination and germinating season (i.e., autumn or spring), between pre-dispersal seed germination and habitat type (i.e., S, GD, and SD), and between pre-dispersal seed germination and life form (i.e., AH, S, SS, and ST). One-way ANCOVAs were used to analyze differences in final survival percentage and dry mass at the 4-leaf stage of S1, S2, and S3 seedlings of Salsola brachiata, with initial seedling size (i.e., dry mass) as a covariate to minimize the effects of variation in initial size among seedlings on survivorship. All data analyses were performed with the software SPSS 19.0 (SPSS Inc., Chicago, IL, United States). Values are means ± 1SE.
Results
Autumn survey of pre-dispersal seed germination
In autumn 2021, we observed 69 species in 25 genera of Amaranthaceae in the three habitats (S, 37 species in 17 genera; GD, 51 species in 19 genera; and SD, 51 species in 21 genera). In the three habitats, trends for number of species of each life form were the same. Frequency of both annual herb (AH) and shrub (SH) life forms was the highest, and the rank order of life forms [i.e. annual herb (AH), shrub (SH), semi-shrub (SS), or small tree (ST)] in the three habitats was: S, [AH (25) > SS (9) > ST (2) > SH (1)]; GD, [AH (34) > SS (13) > SH (3) > ST (1)]; and SD, [AH (32) > SS (15) > SH (3) > ST (1)]. In autumn 2021, no species exhibited pre-dispersal seed germination in any of the three habitat types (Table 1).
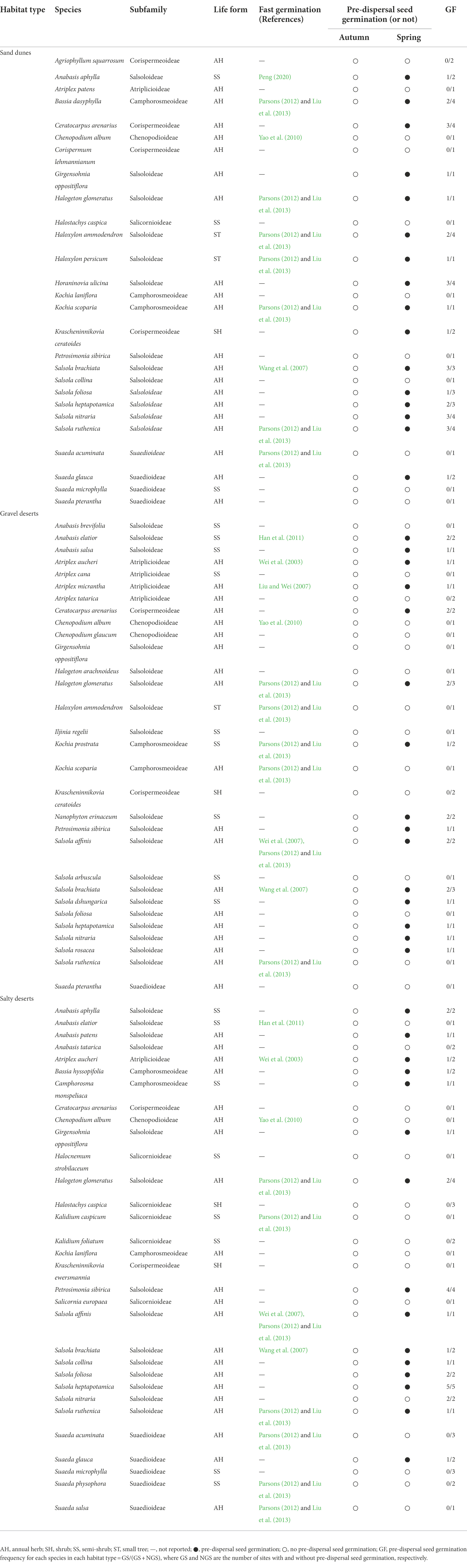
Table 1. Pre-dispersal seed germination (or not) in Amaranthaceae species (in various subfamilies of Amaranthaceae) in the cold deserts of northern Xinjiang in both autumn 2021 and spring 2022 and reports in the literature of very fast germination occurring in the species of Amaranthaceae surveyed in this study.
Spring survey of pre-dispersal seed germination
At the 12 sites in early spring 2022, we found 52 species in 23 genera in 5 subfamilies of Amaranthaceae: S, 27 species in 17 genera; GD, 30 species in 14 genera; and SD, 31 species in 17 genera. Thirty-three of the 52 species (63.5%) were in subfamily Salsoloideae. Trends for number of species for each life form in S, GD, and SD in spring 2022 were the same as those in autumn 2021.
In early spring 2022, when the snow was melting, 30 species (57.7%) exhibited pre-dispersal seed germination (Table 1; Figure 2). The number of species with pre-dispersal seed germination in S, GD, and SD was 16 (59.3%), 15 (50.0%) and 15 (48.4%), respectively (Table 1; Figure 2). Genera with highest number of species with pre-dispersal seed germination were Salsola (9), Anabasis (3), and Atriplex (3) (Figure 3A). In early spring, the number of species with pre-dispersal seed germination varied with life form: annuals (66.67%) > small shrubs (23.33%) > small trees (6.67%) > shrubs (3.33%) (Figure 3B). Thirteen species (43.33%) with pre-dispersal seed germination were found in two or more habitats (Figure 3C): S, 8 (50.0%); GD, 6 (40.0%); and SD, 5 (33.3%) (Table 1).
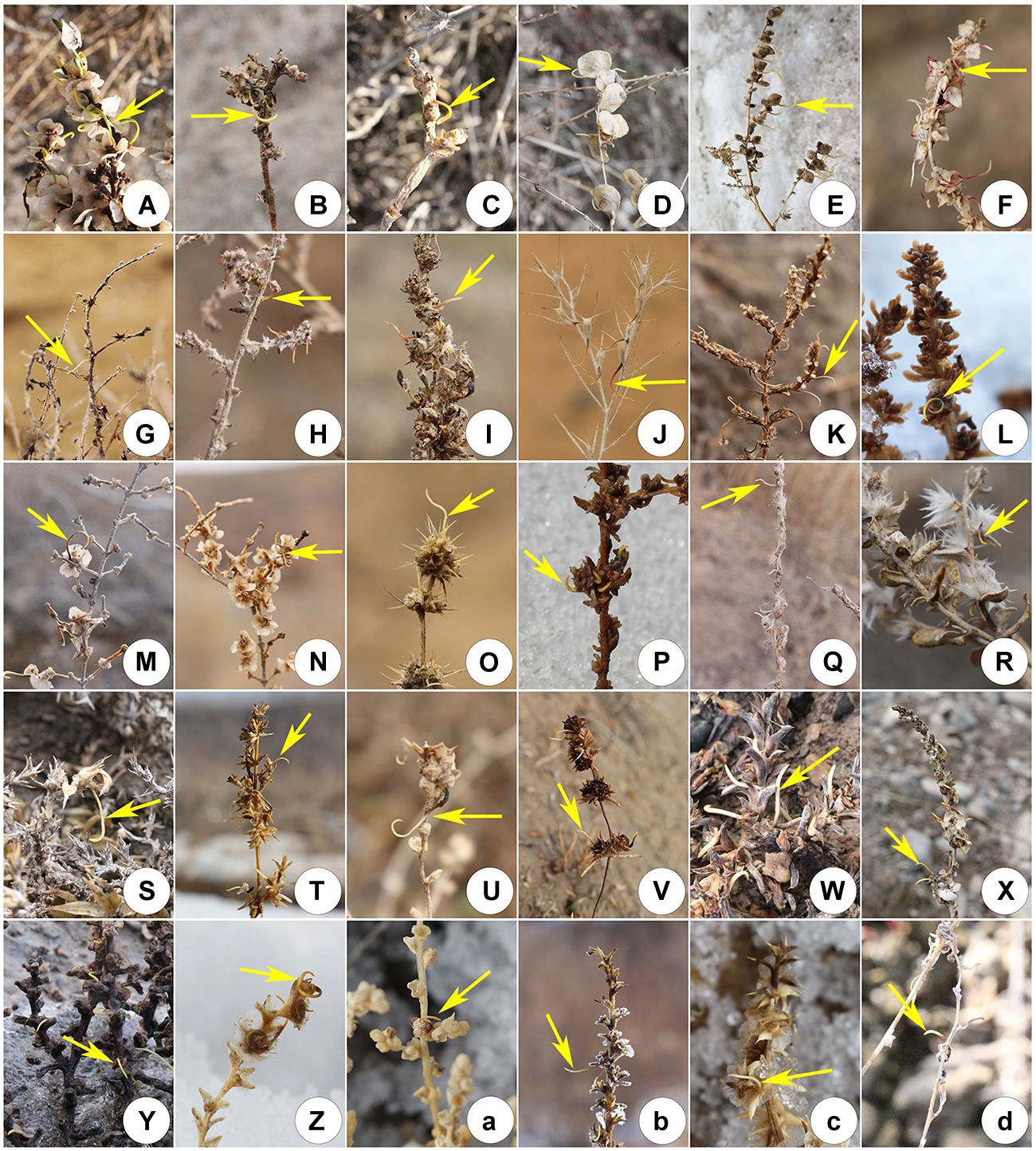
Figure 2. Pre-dispersal seed germination of 30 Amaranthaceae species in cold deserts of northern Xinjiang during snow-melting in early spring 2022. (A) Anabasis aphylla; (B) A. elatior; (C) A. salsa; (D) Atriplex aucheri; (E) A. micrantha; (F) A. patens; (G) Bassia dasyphylla; (H) B. hyssopifolia; (I) Camphorosma monspeliaca; (J) Ceratocarpus arenarius; (K) Girgensohnia oppositiflora; (L) Halogeton glomeratus; (M) Haloxylon ammodendron; (N) H. persicum; (O) Horaninovia ulicina; (P) Kochia prostrata; (Q) K. scoparia; (R) Krascheninnikovia ceratoides; (S) Nanophyton erinaceum; (T) Petrosimonia sibirica; (U) Salsola affinis; (V) S. brachiata; (W) S. collina; (X) S. dshungarica; (Y) S. foliosa; (Z) S. heptapotamica; (a) S. nitraria; (b) S. rosacea; (c) S. ruthenica; (d) Suaeda glauca.
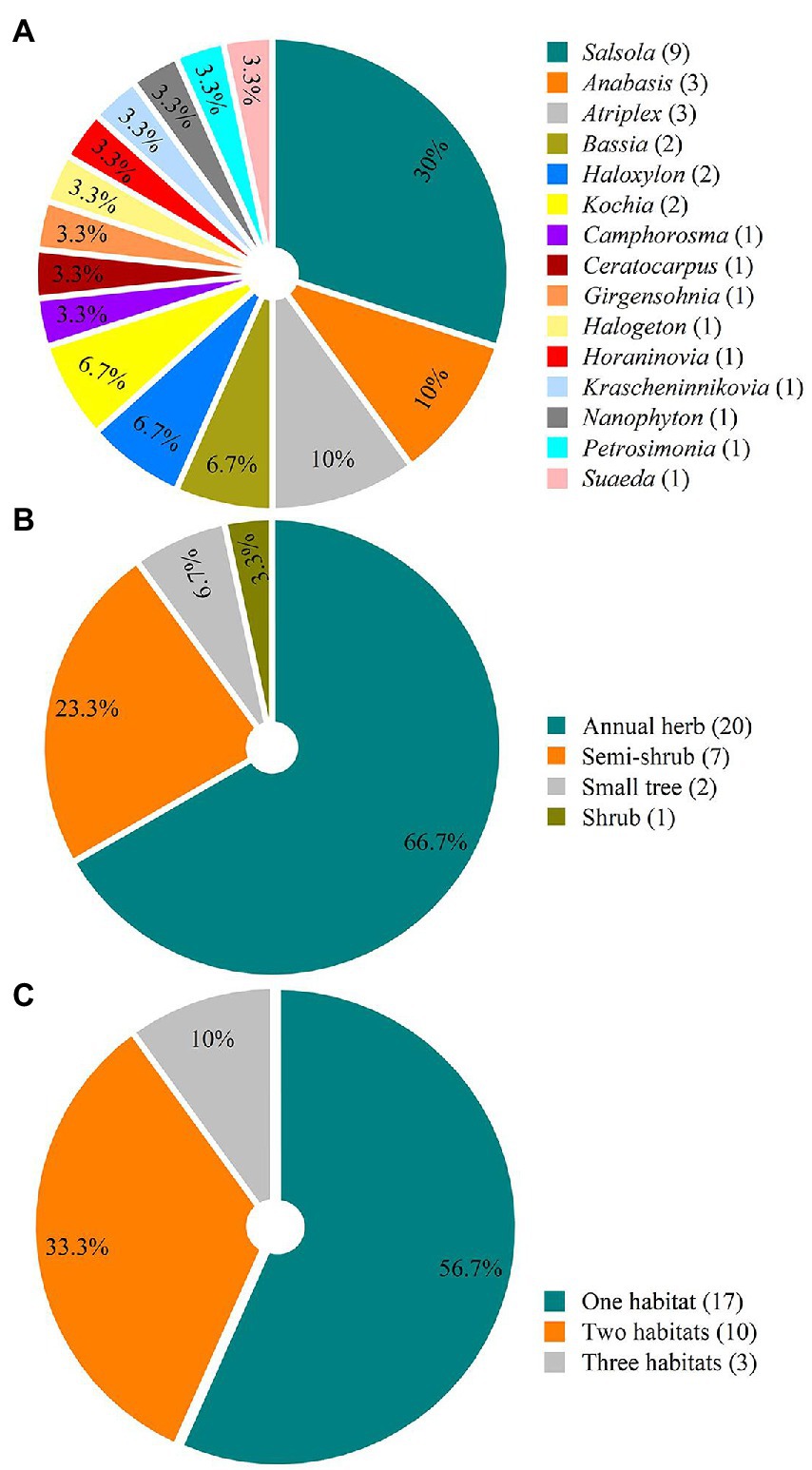
Figure 3. Percentage of each genus (A), life form (B), and habitat type (C) of 30 Amaranthaceae species with the pre-dispersal seed germination in spring 2022 in the cold deserts of northern Xinjiang. Number in parenthesis beside each legend indicates number of species.
Correlation analysis also showed that the relationship between pre-dispersal seed germination and germination season (autumn vs. spring) was significantly negative (r = −0.98, p < 0.05). Pre-dispersal seed germination was found in the three habitat types (S, GD, and SD) and in the four life forms (AH, S, SS, and ST). The relationships between pre-dispersal seed germination and habitat type and between pre-dispersal seed germination and life form were nonsignificantly negative (r = −0.01, p = 1.00) and positive (r = 0.01, p = 0.91), respectively.
Stages in development of pre-dispersal germinated seedlings
Development of seedlings from the pre-dispersal germinated seeds for each of the 30 species was similar and can be divided into four stages: (I) the radicle initially emerged between the bracteoles; (II) the radicle completed emergence and hypocotyl emerged between the bracteoles; (III) the cotyledons mostly emerged through the bracteoles; and (IV) seedlings dropped to the soil surface. The development stages of seedlings from pre-dispersal germinated seeds of Anabasis elatior, Atriplex patens, Kochia prostrata, and Salsola heptapotamica are shown in Figure 4.
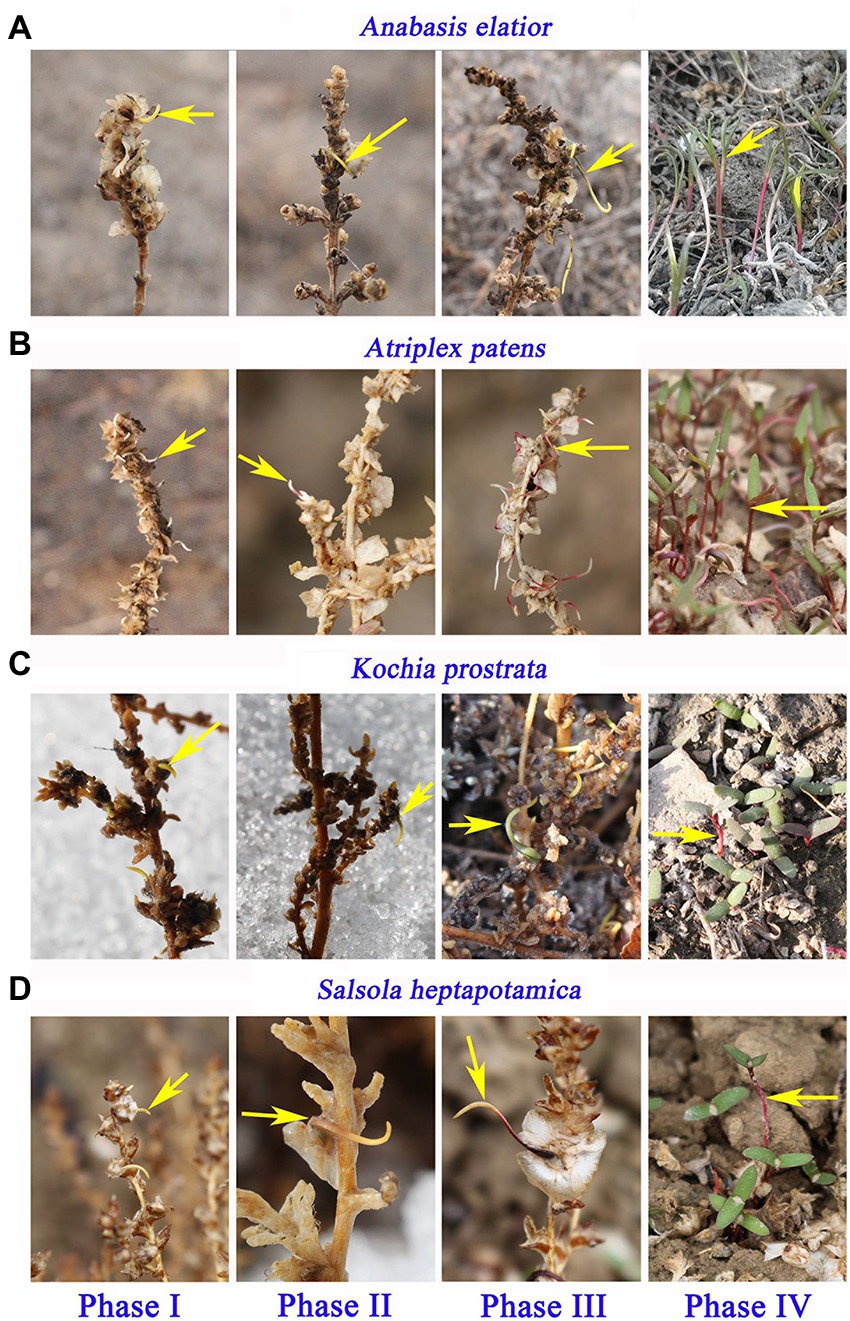
Figure 4. The development of seedlings from pre-dispersal germinated seeds for four Amaranthaceae species [(A) Anabasis elatior (top row), (B) Atriplex patens (second row), (C) Kochia prostrata (third row), and (D) Salsola heptapotamica (bottom row)] in the cold deserts of northern Xinjiang. Phase I, radicle initially emerged from the bracteoles; Phase II, radicle completely emerged and hypocotyl emerged from the bracteoles; Phase III, cotyledons mostly emerged through the bracteoles; and Phase IV, seedlings dispersed to the soil surface.
When the pre-dispersal germinated seedlings fell from the mother plants, their cotyledons were fully expanded, but at this time the cotyledons of the seedlings that germinated on the soil had not unfolded.
Survival and growth of Salsola brachiata seedlings/juveniles
Final survival of S1 seedlings/juveniles (56.7%) was significantly lower than that of S2 (86.0%) and S3 (84.0%), which did not differ significantly from each other (Figure 5A). The final dry mass of the three types of seedlings did not differ significantly for each other (p = 0.19; Figure 5B).
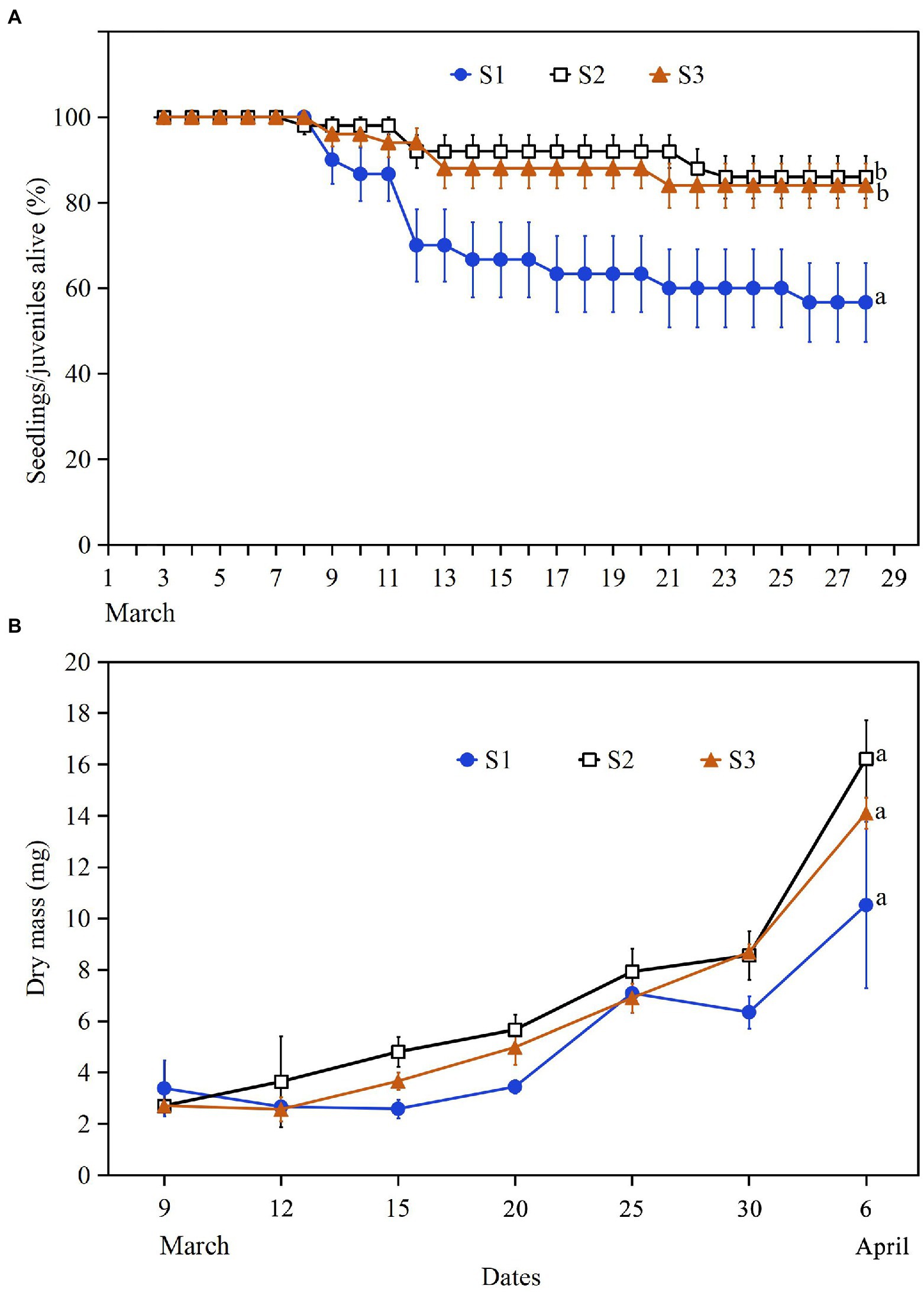
Figure 5. Percentages (mean ± SE) of seedlings/juveniles alive (A) and dry mass of seedlings (B) of Salsola brachiata in spring 2022. S1, seedlings from plant-germinated seeds; S2, seedlings from soil-germinated seeds that dispersed before snow in autumn 2021; S3, seedlings from soil-germinated seeds that dispersed in winter 2021 and at snowmelt in early spring 2022. Different lowercase letters indicate significant differences in final percentage survival (A) or final dry mass (B) of S1, S2, and S3 seedlings.
Discussion
Our prediction that pre-dispersal seed germination is limited to the snowmelt period in spring was supported. At the time of snowmelt in spring, pre-dispersal germination of seeds of various species of Amaranthaceae was documented in sand dunes, gravel deserts, and salt deserts, but no pre-dispersal germination was found in these habitats in autumn. To our knowledge this is the first report of pre-dispersal seed germination in Amaranthaceae or of early survival of seedlings/juveniles of Amaranthaceae with pre-dispersal germination.
Pre-dispersal seed germination as reported here for various species of Amarnathaceae is not vivipary sensu stricto. That is, it is not (sexually-based) true vivipary or cryptovivipary such as occurs in mangroves (Goebel, 1905; Guppy, 1906, 1912; Sussex, 1975; Tomlinson, 1986; Elmqvist and Cox, 1996; Leck and Outred, 2008) in which the embryo does not go through a “rest period” between completion of embryogeny and germination. Growth of the embryo in mangroves is continuous into the seedling stage of the life cycle, and the seeds are desiccation intolerant (recalcitrant). On the other hand, seeds of Amaranthaceae in our study are desiccation tolerant (orthodox) in storage behavior. Subbiah et al. (2019) did not list any species of Amaranthaceae in their global list of recalcitrant-seeded species. And pre-dispersal seed germination in the Amaranthaceae species certainly is not (asexually-based) pseudo-vivipary (sensu Elmqvist and Cox, 1996) in which sexual propagules [caryopses (seeds)] in an inflorescence are replaced, in whole or in part, by asexual (vegetative) propagules, as occurs, for example, in various species of grasses and particularly in those growing at high latitudes and altitudes (e.g., Wycherley, 1953; Moore and Doggett, 1976; Lee and Harmer, 1980; Alsos et al., 2013; Lo Medico et al., 2018).
Another term used to refer to germination of sexually-produced seeds while they are still on the mother plant is pre-harvest sprouting (PHS), which is common in seeds (grains) of cereals, i.e., barely, maize, oats, rice, rye, sorghum, and wheat (all of which have orthodox storage behavior), and causes much economic loss to farmers in many parts of the world. PHS refers to a phenomenon in which developing grains germinate viviparously (precociously) on the mother plant prior to harvest. The primary cause of PHS is lack of dormancy of the grain under humid/wet environmental conditions (e.g., Eyster, 1931; Neill et al., 1987; Lund et al., 2001; Paulsen and Auld, 2004; Rodríguez et al., 2015; Singh et al., 2021; Sohn et al., 2021). However, whereas the cereal grains that germinate viviparously do not go through a rest period before they germinate, seeds of the Amaranthaceae species in our study go through an extended autumn → late winter/early spring rest period (quiescence, dormancy) before they germinate on the mother plant.
Seeds of some species of Amaranthaceae are nondormant at maturity, e.g., the brown seeds of the dimorphic species Suaeda aralocaspica (Wang et al., 2008). However, even in species with nondormant seeds, no pre-dispersal germination occurs in late autumn. An important reason for lack of pre-dispersal (and even post-dispersal) germination in autumn in the cold deserts of Central Asia is lack of rainfall during autumn (Lu et al., 2022). Another reason for lack of germination in autumn is that freshly-matured seeds have physiological dormancy, e.g., two of the three seed morphs of Atriplex centralasiatica (Wang et al., 2020), two of three seed morphs of Salsola affinis (Wei et al., 2008), one of four seed morphs of S. brachiata (Baskin et al., 2014), the black seed morph of the dimorphic Suaeda aralocaspica (Wang et al., 2008), and both the two basicarps and the aerial dispersal units of Ceratocarpus arenarius (Lu et al., 2013).
Pre-dispersal germination in spring requires that some seeds remain on the mother plant over winter. Although seeds may be dispersed in mid-to late autumn, many are not dispersed, probably (at least in part) because they become covered by snow. Thus, two cohorts of seeds from the same mother plants are present in spring: seeds dispersed to the soil surface and covered by snow and seeds not dispersed and still attached to dead stems of mother plants that are covered by snow.
The number of plants with seeds remaining on them the following spring varied with the life form and habitat type (Table 1). For example, in the S habitat type only a few seeds remained on the small trees (Haloxylon ammodendron and Haloxylon persicum) and the shrub (Krascheninnikovia ceratoides), while many seeds remained on the annuals (Ceratocarpus arenarius and Salsola brachiata) and semi-shrubs (Anabasis aphylla and Suaeda microphylla). Likewise, for the same species the number of seeds remaining on the plants varied in habitat types. For example, in Haloxylon ammodendron some seeds of this species remained on the mother plants in S, while no seeds remained on the plants in GD.
The seeds attached to the dead stems of mother plants are the first ones exposed to increasing temperatures in spring (and to high moisture levels due to melting snow), and they germinate rapidly. Nondormant seeds that can germinate in <24 h from the beginning of imbibition to radicle emergence are said to be “very fast germinating” (Parsons, 2012; Liu et al., 2013; Parsons et al., 2014; Kadereit et al., 2017). At maturity, seeds with very fast germination are characterized by fully developed and often chlorophyllous embryos, and they occur in taxa whose fresh seeds are either nondormant or have nondeep physiological dormancy (Parsons, 2012; Liu et al., 2013). Liu et al. (2013) used the term “cryptoviviparous-like” to describe seeds with very fast germination. Based on available literature, very fast germination is mainly found in stressful, highly dynamic and pioneer habitats, where it reduces chances of seedling mortality (Karrenberg et al., 2002; Gutterman, 2003). Of the 49 species reported to have very fast germinating seeds, 27 (55%) are in the Amaranthaceae (Parsons, 2012; Parsons et al., 2014; Kadereit et al., 2017). Thirteen of the 30 species with pre-dispersal germination in our study have been reported to have very fast germination (Table 1). Five species (i.e., Chenopodium album, Kalidium caspicum, Suaeda acuminata, Suaeda physophora, and Suaeda salsa) have been reported to have very fast germination, but we did not find pre-dispersal germination for them in spring 2022. One possible reason is that the plants of these five “winter-upright” species are too tall to have good snow cover, and thus seeds did not have the high moisture level required for germination.
More plants of S. brachiata from the post-dispersal germinated seeds survived to the four-leaf stage than those from the pre-dispersal germinated seeds. In the cold deserts of Central Asia, rainfall is highly variable among seasons and years, but generally rainfall is higher in spring than in autumn (Wang et al., 2006). Further, snowmelt increases water availability in spring, which promotes seedling establishment (Lu et al., 2014a,b; Bie et al., 2016). It seems reasonable that pre-dispersal germination in a low rainfall year would result in earlier establishment/growth of seedlings from pre-dispersal than those from post-dispersal germinating seeds. Further, although many seedlings from pre-dispersal germinating seeds die, their seed production per plant could be higher than that of those from post-dispersal germinating seeds (sensu Wang et al., 2010). The lower survival percentages of plants from pre-dispersal than post-dispersal germinating seeds suggests that pre-dispersal germination may be maladaptive, but more studies are needed.
In conclusion, the results from our study raise several questions. For example, are the seeds with and without pre-dispersal germination the same size and have the same degree of dormancy? In the achene-dimorphic species Grindelia squarrosa (Asteraceae), only disc achenes had pre-dispersal germination, i.e., no ray achenes germinated; disc achenes are less dormant than ray achenes (Pliszki and Górecki, 2021). Additionally, what might be the effect of climate change on the life history, ecology, and demography on Amaranthaceae species with pre-dispersal germination in the cold deserts, especially with regard to amount and frequency of precipitation? These questions need to be addressed by future research.
Data availability statement
The original contributions presented in the study are included in the article, further inquiries can be directed to the corresponding author.
Ethics statement
No specific permits were required for the described field studies. The locations are not privately-owned or protected in any way, and the field studies did not involve endangered or protected species.
Author contributions
JL and DT conceived and designed the experiments. JL, WL, and JH performed data collection. JL analyzed the data. JL, DT, CB, and JB wrote the manuscript. All authors contributed to the article and approved the submitted version.
Funding
This research was supported in part by the Excellent Youth Foundation of Natural Science Foundation of Xinjiang Uygur Autonomous Region of China (2021D01E21), the National Natural Science Foundation of China (32160265, U1803331, and 32071668), the Third Xinjiang Scientific Expedition Program (2022xjkk1201 and 2022xjkk1203) and Training Project for Excellent Youth Science and Technology Scholars of Urumqi Science and Technology Talent Foundation of China.
Acknowledgments
We thank Xuelan Zhou and Jiaben Jia for help in collecting samples and the National Meteorological Information Center, China Meteorological Administration for providing the temperature data.
Conflict of interest
The authors declare that the research was conducted in the absence of any commercial or financial relationships that could be construed as a potential conflict of interest.
Publisher’s note
All claims expressed in this article are solely those of the authors and do not necessarily represent those of their affiliated organizations, or those of the publisher, the editors and the reviewers. Any product that may be evaluated in this article, or claim that may be made by its manufacturer, is not guaranteed or endorsed by the publisher.
References
Alsos, I. G., Müller, E., and Eidesen, P. B. (2013). Germinating seeds or bulbils in 87 of 113 arctic species indicate potential for ex situ seed bank storage. Polar Biol. 36, 819–830. doi: 10.1007/s00300-013-1307-7
Baskin, J. M., and Baskin, C. C. (1972). Influence of germination date on survival and seed production in a natural population of Leavenworthia stylosa. Amer. Midl. Nat. 88, 318–323. doi: 10.2307/2424357
Baskin, J. M., Lu, J. J., Baskin, C. C., Tan, D. Y., and Wang, L. (2014). Diaspore dispersal ability and degree of dormancy in heteromorphic species of cold deserts of Northwest China: a review. Perspect. Plant Ecol. Evol. System. 16, 93–99. doi: 10.1016/j.ppees.2014.02.004
Bie, B., Zhou, X., and Zhang, Y. (2016). Effects of snow cover on seed germination of ten desert plant species. Chin. J. Ecol. 35, 2348–2354. (in Chinese with English abstract). doi: 10.13292/j.1000-4890.201609.033
Commissione Redactorum Florae Xinjiangensis (1994). Flora Xinjiangensis. Urumqi: Xinjiang Science & Technology & Hygiene Publishing House. (in Chinese)
Donohue, K., Rubio de Casas, R., Burghardt, L., Kovach, K., and Willis, C. G. (2010). Germination, postgermination adaptation, and species ecological ranges. Annu. Rev. Ecol. Evol. Syst. 41, 293–319. doi: 10.1146/annurev-ecolsys-102209-144715
Elmqvist, T., and Cox, P. A. (1996). The evolution of vivipary in flowering plants. Oikos 77, 3–9. doi: 10.2307/3545579
Fang, X., Chen, Z., Guo, X., Zhu, S., Liu, T., Li, C., et al. (2019). Impacts and uncertainties of climate/CO2 change on net primary productivity in Xinjiang, China (2000-2014): a modelling approach. Ecol. Model. 408:108742. doi: 10.1016/j.ecolmodel.2019.108742
Goebel, K. E. (1905). Organography of Plants. Part II. Special Organography. Oxford: Clarendon Press.
Guppy, H. B. (1906). Observations of a naturalist in the Pacific between 1896 and 1899. Part II. Plant dispersal. New York: Macmillan and Company.
Gutterman, Y. (2003). The inhibitory effect of Negev Desert loess soil on the fast germinating caryopses of Schismus arabicus (Poaceae). J. Arid Environ. 54, 619–631. doi: 10.1006/JARE.2002.1104
Han, J. X., Wei, Y., Yan, C., and An, S. Z. (2011). The vivipary characteristic of Anabasis elatior and its ecological adaptation. Acta Ecol. Sin. 31, 2662–2668. (in Chinese with English abstract).
Huang, G., Li, Y., Mu, X., Zhao, H., and Cao, Y. (2017). Water-use efficiency in response to simulated increasing precipitation in a temperate desert ecosystem of Xinjiang China. J. Arid. Land 9, 823–836. doi: 10.1007/s40333-017-0021-7
Kadereit, G., Newton, R. J., and Vandelook, F. (2017). Evolutionary ecology of fast seed germination – a case study in Amaranthaceae/Chenopodiaceae. Perspect. Plant Ecol. Evol. System. 29, 1–11. doi: 10.1016/j.ppees.2017.09.007
Karrenberg, S., Edwards, P. J., and Kollman, J. (2002). The life history of Salicaceae living in the active zone of floodplains. Freshw. Biol. 47, 733–748. doi: 10.1046/J.1365-2427.2002.00894.X
Leck, M. A., and Outred, H. A. (2008). “Seedlings natural history” in Seedling Ecology and Evolution. eds. M. A. Leck, V. T. Parker, and R. L. Simpson (Cambridge: Cambridge University Press), 17–41.
Lee, J. A., and Harmer, R. (1980). Vivipary, a reproductive strategy in response to environmental stress? Oikos 35, 254–265. doi: 10.2307/3544433
Li, J., Chen, H., and Zhang, C. (2020). Impacts of climate change on key soil ecosystem services and interactions in Central Asia. Ecol. Indic. 116:106490. doi: 10.1016/j.ecolind.2020.106490
Lioubimtseva, E., and Cole, R. (2006). Uncertainties of climate change in arid environments of Central Asia. Rev. Fish. Sci. 14, 29–49. doi: 10.1080/10641260500340603
Liu, K., Baskin, J. M., Baskin, C. C., and Du, G. (2013). Very fast-germinating seeds of desert species are cryptoviparous-like. Seed Sci. Res. 23, 163–167. doi: 10.1017/S0960258513000135
Liu, P. W., and Wei, Y. (2007). Seed dimorphism and germination behavior of Atriplex micrantha, an annual inhabiting Junggar Desert. Acta Ecol. Sin. 27, 4233–4239. (in Chinese with English abstract). doi: 10.3321/j.issn:1000-0933.2007.10.034
Lo Medico, J. M., Rua, G. H., Bonasora, M. G., and Vega, A. S. (2018). Floral reversion and first record of pseudovivipary in some species of Poaceae. Adansonia 40, 59–66. doi: 10.5252/adansonia2018v40a5
Lu, J. J., Dong, W. J., Tan, D. Y., Baskin, C. C., and Baskin, J. M. (2020). Dormancy and germination of the trimorphic achenes of a cold desert annual: spreading the risk over time. AoB Plants 12:plaa056. doi: 10.1093/aobpla/plaa056
Lu, J. J., Tan, D. Y., Baskin, J. M., and Baskin, C. C. (2013). Trade-offs between seed dispersal and dormancy in an amphi-basicarpic cold desert annual. Ann. Bot. 112, 1815–1827. doi: 10.1093/aob/mct240
Lu, J. J., Tan, D. Y., Baskin, J. M., and Baskin, C. C. (2014a). Germination season and watering regime, but not seed morph, affect life history traits in a cold desert diaspore-heteromorphic annual. PLoS One 9:e102018. doi: 10.1371/journal.pone.0102018
Lu, J. J., Tan, D. Y., Baskin, J. M., and Baskin, C. C. (2014b). Two kinds of persistent soil seed banks in an amphi-basicarpic cold desert annual. Seed Sci. Res. 24, 293–300. doi: 10.1017/S0960258514000270
Lu, J. J., Tan, D. Y., Baskin, C. C., and Baskin, J. M. (2017). Role of indehiscent pericarp in formation of soil seed bank in five cold desert Brassicaceae species. Plant Ecol. 218, 1187–1200. doi: 10.1007/s11258-017-0760-8
Lu, J. J., Tan, D. Y., Baskin, C. C., and Baskin, J. M. (2022). “Effects of climate change on plant regeneration from seeds in the cold deserts of Central Asia” in Plant Regeneration from Seeds: A Global Warming Perspective. eds. C. C. Baskin and J. M. Baskin (San Diego: Elsevier, Academic Press), 33–46.
Lund, G. D., Major, B. J., Kettlewell, P. S., and Scott, R. K. (2001). Mechanisms leading to excess alpha-amylase activity in wheat (Triticum aestivum, L.) grain in the U.K. J. Cereal Sci. 33, 313–329. doi: 10.1006/jcrs.2001.0369
Maleki, K., Baskin, C. C., Baskin, J. M., Kiani, M., Alahdadi, I., and Soltani, E. (2022). Seed germination thermal niche differs among nine populations of an annual plant: a modelling approach. Ecol. Evol. 12:e9240. doi: 10.1002/ece3.9240
Mao, Z. M. (1994). Flora of Xinjiang, 2. Urümqi: Xinjiang, Science, Technology & Public Health Press. (in Chinese)
Moore, D. M., and Doggett, M. C. (1976). Pseudo-vivipary in Fuegian and Falkland islands grasses. Antarct. Surv. Bull. 43, 103–110.
Neill, S. J., Horgan, R., and Rees, A. F. (1987). Seed development and vivipary in Zea mays L. Planta 171, 358–364. doi: 10.1007/BF00398681
Parsons, R. F. (2012). Incidence and ecology of very fast germination. Seed Sci. Res. 22, 161–167. doi: 10.1017/S0960258512000037
Parsons, R. F., Vandelook, F., and Janssens, S. B. (2014). Very fast germination: additional records and relationship to embryo size and phylogeny. Seed Sci. Res. 24, 159–163. doi: 10.1017/s096025851400004x
Paulsen, G. M., and Auld, A. S. (2004). “Preharvest sprouting of cereals” in Handbook of Seed Physiology: Applications to Agriculture. eds. R. L. Benech-Arnold and R. A. Sánchez (New York: Food Products Press), 199–219.
Peng, M. R. (2020). Response of Seed Germination Process of Desert Plant Anabasis aphylla to Low Temperature. master’s thesis. Shihezi: Shihezi University. (in Chinese with English abstract). doi: 10.27332/d.cnki.gshzu.2020.000426
Pliszki, A., and Górecki, A. (2021). First observations of true vivipary in Grindelia squarrosa (Asteraceae). Biologia 76, 1147–1151. doi: 10.1007/S11756-021-00713-0
Postma, F. M., and Ågren, J. (2022). Effects of primary seed dormancy on lifetime fitness of Arabidopsis thaliana in the field. Ann. Bot. 129, 795–807. doi: 10.1093/aob/mcac010
Rodríguez, M. V., Barrero, J. M., Corbineau, F., Gubler, F., and Benech-Arnold, R. L. (2015). Dormancy in cereals (not too much, not so little): about the mechanisms behind this trait. Seed Sci. Res. 25, 99–119. doi: 10.1017/S0960258515000021
Shi, Y. G., and Sun, Z. B. (2008). Climate characteristics of atmospheric precipitable water over Xinjiang and its variation. J. Desert Res. 28, 519–525. (in Chinese with English abstract). doi: 10.3724/SP.J.1011.2008.00323
Singh, C., Kamble, U. R., Gupta, V., Singh, G., Sheoran, S., Gupta, A., et al. (2021). Pre-harvest sprouting in wheat: current status and future prospects. J. Cereal Res. 13, 1–22. doi: 10.25174/2582-2675/2021/114484
Sohn, S. I., Pandian, S., Kumar, T. S., Zoclanclounon, Y. A. B., Muthuramalingam, P., Shilpha, J., et al. (2021). Seed dormancy and pre-harvest sprouting in rice-An updated overview. Int. J. Mole. Sci. 22:11804. doi: 10.3390/ijms222111804
Soriano, A. (1983). “Deserts and semi-deserts of Patagonia” in Ecosystems of the World 5. Temperate Deserts and Semi Deserts. ed. N. E. West (Amsterdam: Elsevier), 423–460.
Su, H. C., Shen, Y. P., Han, P., Li, J., and Lan, Y. C. (2007). Precipitation and its impact on water resources and ecological environment in Xinjiang region. J. Glaciol. Geocryol. 29, 343–350. (in Chinese with English abstract). doi: 10.3969/j.issn.1000-0240.2007.03.002
Subbiah, A., Ramdhani, S., Pammenter, N. W., Macdonald, A. H. H., and Sershen, (2019). Towards understanding the incidence and evolutionary history of seed recalcitrance: an analytical review. Perspect. Plant Ecol. Evol. System. 37, 11–19. doi: 10.1016/j.ppees.2019.01.001
Sussex, I. (1975). Growth and metabolism of the embryo and attached seedling of the viviparous mangrove, Rhizophora mangle. Amer. J. Bot. 62, 948–953. doi: 10.1002/j.1537-2197.1975.tb14135.x
Walter, H., and Box, E. O. (1983). “Caspian lowland biome” in Ecosystems of the World 5. Temperate Deserts and Semi-Deserts. ed. N. E. West (Amsterdam: Elsevier), 9–41.
Wang, Z., Baskin, J. M., Baskin, C. C., Yang, X., Liu, G., and Huang, Z. Y. (2020). Dynamics of diaspore and germination stages of the life history of an annual diaspore-trimorphic species in a temperate salt desert. Planta 251:87. doi: 10.1007/s00425-020-03380-8
Wang, L., Huang, Z. Y., Baskin, C. C., and Baskin, J. M. (2008). Germination of dimorphic seeds of the desert annual halophyte Suaeda aralocaspica (Chenopodiaceae), a C4 plant without kranz anatomy. Ann. Bot. 102, 757–769. doi: 10.1093/aob/mcn158
Wang, X. Q., Jiang, J., Wang, Y. C., Luo, W. L., Song, C. W., and Chen, J. (2006). Responses of ephemeral plant germination and growth to water and heat conditions in the southern part of Gurbantunggut Desert. Chin. Sci. Bull. 51, S110–S116. doi: 10.1007/s11434-006-8214-z
Wang, A. B., Tan, D. Y., Baskin, C. C., and Baskin, J. M. (2010). Effect of seed position in spikelet on life history of Eremopyrum distans (Poaceae) from the cold desert of Northwest China. Ann. Bot. 106, 95–105. doi: 10.1093/aob/mcq089
Wang, H. F., Wei, Y., and Huang, Z. Y. (2007). Seed polymorphism and germination of Salsola bracchita [brachiata], a dominant desert annual inhabiting Junggar Basin of Xinjiang. China. J. Plant Ecol. (Chinese Version) 31, 1046–1053. (in Chinese with English abstract). doi: 10.17521/cjpe.2007.0132
Wei, Y., Dong, M., and Huang, Z. Y. (2007). Seed polymorphism, dormancy and germination of Salsola affinis (Chenopodiaceae), a dominant desert annual inhibiting the Junggar basin of Xinjiang, China. Aust. J. Bot. 55, 464–470. doi: 10.1071/BT06016
Wei, Y., Dong, M., Huang, Z., and Tan, D. (2008). Factors influencing seed germination of Salsola affinis (Chenopodiaceae), a dominant annual halophyte inhabiting the deserts of Xinjiang, China. Flora 203, 134–140. doi: 10.1016/j.flora.2007.02.003
Wei, Y., Yan, C., and Yin, L. (2003). Seed polymorphism and ecotype of Atriplex aucheri. Acta Bot. Boreal.-Occident. Sin. 23, 485–487. (in Chinese with English abstract). doi: 10.3321/j.issn:1000-4025.2003.03.024
West, N. E. (1983). “Comparisons and contrasts between the temperate deserts and semi-deserts of three continents” in Ecosystems of the World 5. Temperate Deserts and Semi-Deserts. ed. N. E. West (Amsterdam: Elsevier), 461–472.
Wycherley, P. R. (1953). The distribution of the viviparous grasses in Great Britain. J. Ecol. 41, 275–288. doi: 10.2307/2257041
Xinjiang General Exploration Team of Chinese Academy of Sciences (CAS), and Institute of Botany of CAS (1978). Vegetation of Xinjiang and its Utilization. Beijing: Science Publishing House. (in Chinese)
Yao, S., Lan, H., and Zhang, F. (2010). Variation of seed heteromorphism in Chenopodium album and the effect of salinity stress on the descendants. Ann. Bot. 105, 1015–1025. doi: 10.1093/aob/mcq060
Keywords: cold desert, maladaptive, pre-dispersal seed germination, snowmelt, unpredictable-rainfall environment
Citation: Lu J, Liu W, Han J, Tan D, Baskin CC and Baskin JM (2022) Non-viviparous pre-dispersal seed germination in Amaranthaceae in the cold deserts of Central Asia. Front. Ecol. Evol. 10:1047330. doi: 10.3389/fevo.2022.1047330
Edited by:
Dechang Cao, Kunming Institute of Botany (CAS), ChinaReviewed by:
Kun Liu, Lanzhou University, ChinaLei Wang, Xinjiang Institute of Ecology and Geography (CAS), China
Anurag Dhyani, Jawaharlal Nehru Tropical Botanic Garden and Research Institute, India
Copyright © 2022 Lu, Liu, Han, Tan, Baskin and Baskin. This is an open-access article distributed under the terms of the Creative Commons Attribution License (CC BY). The use, distribution or reproduction in other forums is permitted, provided the original author(s) and the copyright owner(s) are credited and that the original publication in this journal is cited, in accordance with accepted academic practice. No use, distribution or reproduction is permitted which does not comply with these terms.
*Correspondence: Dunyan Tan, tandunyan@xjau.edu.cn