Alleviation of heavy metal stress and enhanced plant complex functional restoration in abandoned Pb–Zn mining areas by the nurse plant Coriaria nepalensis
- 1Yunnan Key Laboratory for Plateau Mountain Ecology and Restoration of Degraded Environments, School of Ecology and Environmental Sciences, Yunnan University, Kunming, China
- 2Yunnan International Joint Research Center of Plateau Lake Ecological Restoration and Watershed Management, Yunnan University, Kunming, China
Heavy metal pollution caused by mining has been a topic of concern globally because it threatens ecological functions and human health. Nearly all current remediation strategies take into account only such short-term issues as how to reduce or stabilize the content of heavy metals in soil, how to reduce the toxicity of heavy metals, and how to preserve water, soil and nutrients. However, little attention is paid to such long-term issues as whether plants can survive, whether communities can be stabilized, and whether ecosystem functions can be restored. Therefore, improving plant diversity and community stability are key aspects of improved mine restoration. To explore the possibility of reconstructing plant complexes in mining areas, the local nurse plant Coriaria nepalensis was selected as the research object for a study in the Huize Pb–Zn mining area of southwest China. C. nepalensis could increase the contents of nutrient elements (C, N, and P), reduce the contents of heavy metals (Mn, Cu, Zn, Cd, and Pb), and strengthen the plant complex functions (diversity, functional traits, and complex biomass) in its root zone. In general, C. nepalensis can form fertility islands (survival islands) in mining areas, which facilitate the colonization and success of additional less stress-resistant species. We propose C. nepalensis as a key species for use in restoration based on its ability to restore ecosystem functions under extremely stressful conditions. We encourage combination of C. nepalensis with other nurse plants to reinforce the rehabilitation of ecosystem functions.
Highlights
– Coriaria nepalensis formed fertility islands (survival islands) in abandoned Pb–Zn mining areas.
– Coriaria nepalensis increased the contents of nutrient elements (C, N, and P) in its root zone.
– Coriaria nepalensis reduced the contents of heavy metals (Mn, Cu, Zn, Cd, and Pb) in its root zone.
– Coriaria nepalensis strengthened the plant complex functions (diversity, functional traits, and complex biomass) in its root zone.
– Coriaria nepalensis weakened the toxicity of heavy metals and the intensity of competition among plants.
Introduction
The mining of metals creates abandoned land featuring severe abiotic stresses imposed by high concentrations of trace elements, high conductivity, physical and chemical instability, and scant soil (Coelho et al., 2011; Li et al., 2022). In addition, overexploitation has caused tremendous harm to biodiversity and ecosystems (i.e., land and food security, soil quality, water quality, vegetation, and ecosystem services; Hossain et al., 2013; Gosain et al., 2015; Haddaway et al., 2019). Furthermore, heavy metals diffuse along with runoff, thus threatening the soil health and production of humans (Yang et al., 2012; Wang et al., 2020). Excessive exposure to heavy metals is associated with a variety of ailments (Munford et al., 2020). More importantly, land pollution and degradation caused by mining as well as by other anthropogenic activities have expanded worldwide, and the ecological restoration of abandoned lands has become an urgent environmental problem (Ilunga et al., 2015; Liu and Liu, 2020).
The current techniques for rehabilitation of abandoned mining areas may be divided into three categories: physical/mechanical, chemical, and biological methods. The physical/mechanical remediation method is based on earthworks and strives to return the post-mining land to its previous state using practices such as tilling, classifying, leveling, and topsoil replacement (Seenivasan et al., 2015). However, this method is expensive, and it is only suitable for economically developed areas (Festin et al., 2019). The chemical method involves removing excess heavy metals and hazardous chemicals from the soil and adjusting the soil pH (Mensah, 2015). However, chemical reagents are costly, require a vast group of professional technicians, and cause secondary pollution to the soil, so they cannot be utilized on a broad scale (Wu et al., 2010). Biological methods take advantage of the extraction or purifying functions of organisms. The most commonly used biological method is phytoextraction (Bolan et al., 2011), which reduces contamination levels in soil by extracting, translocating, and immobilizing heavy metals through hyperaccumulator plants. Compared to the two aforementioned techniques, phytoextraction has the advantages of in situ remediation, low cost, environmental friendliness, and suitability for large polluted areas (Teixeira et al., 2011). However, although phytoextraction has been touted as a viable method for treating abandoned mining areas, few studies have demonstrated that planting hyperaccumulative plants for mine waste restoration can produce a long-term plant community (Wu et al., 2013; Li and Huang, 2015).Overall, nearly all of these remediation strategies take into account only such short-term issues as how to reduce or stabilize the content of heavy metals in soil, how to reduce the toxicity of heavy metals, and how to preserve water, soil and nutrients. However, little attention is paid to such long-term issues as whether plants can survive, whether communities can be stabilized, and whether ecosystem functions can be restored.
Developing methods to establish stable plant complexes (patches) and prevent the spread of pollution is essential for mine restoration (Köbel et al., 2021; Zeidler et al., 2021). Several studies (Wang et al., 2014b; Jia et al., 2020; Bateman et al., 2021) have revealed that transplanting dominant plants to form new plant complexes may produce a series of benefits to ecosystem functions during mining area rehabilitation, but this process requires sustained professional program management, and the plant complexes cannot develop through succession. In other words, selection of spontaneous plant species is the key to successful restoration of mined land (Loreau and de Mazancourt, 2013). The ideal characteristics for species selection are an ability to establish and grow rapidly on barren substrates (Martínez-Fernández et al., 2011). Pioneer plant species, although very effective at colonizing a barren environment, do not necessarily promote the establishment of other plant species or improve soil conditions (Lei and Duan, 2008; Parraga-Aguado et al., 2013). Nurse plant species are stress-resistant plants that can thrive in difficult conditions (e.g., severely polluted soil, degraded soil, drought, and extreme cold) and establish small islands around their habitats, allowing less stress-tolerant plants to survive and develop beneath their canopies in harsh conditions with abiotic pressures (Padilla and Pugnaire, 2006; Saikia and Khan, 2012; Wang et al., 2014a). Hooper et al. (2005) suggested that necessary ecosystem functions should be promoted by selecting and combining nurse plant species, facilitating the establishment of other plant species and ultimately promoting plant–soil feedbacks. It is critical to search for native nurse plant species and research their roles (including high accumulation of soil organic carbon and nutrients, reducing soil metal concentrations, and improving community stability) in the establishment of plant patches, which are important for the recovery of abandoned mining areas.
According to reports, the total area of land resources lost by mining in the world has exceeded 20 million ha (Liu et al., 2018). In China, more than 118,000 mines are in operation, and the total volume of mine waste produced since 2009 has surpassed 10 billion tons (Pan et al., 2014). There are currently about 12,000 tailings ponds, which cover a significant area of land, including land in agricultural and forest regions (Yin et al., 2011). Uncontrolled mining has wreaked damage on local soil resources and polluted the ecosystem (Risueno et al., 2020b). The Huize Pb–Zn Mining District (Yunnan Province) has been an important metalliferous area in southwestern China since the Ming Dynasty, and conventional smelting processes have resulted in heavy metal contamination of the surrounding soil (Li et al., 2019). We discovered the nurse plant Coriaria nepalensis Wall. (Coriariaceae) (Awasthi et al., 2022a), which may help to alleviate the heavy metal pollution problem in this mining area. C. nepalensis is a common native shrub species of Yunnan Province that grows between 1200 and 2500 m a.s.l. (Awasthi et al., 2022b). A previous study (Mourya et al., 2019) showed that C. nepalensis could accumulate nutrients and thus aid the colonization of additional plants and contribute to the recovery of degraded forest ecosystems in the central Himalaya. To explore the role of C. nepalensis in the abandoned land in the Pb–Zn mining area, we established sample sites at three distinct locations in the mine. More specifically, we assessed the impacts of C. nepalensis on soil fertility, heavy metal concentration, plant diversity, functional diversity, and community productivity; analyzed whether C. nepalensis can form “survival islands” under extreme conditions; and explored the mechanisms by which C. nepalensis influences soil properties and plant complexes. Our results provide information that is useful for restoring polluted and degraded areas, such as tailings, abandoned mines, and quarries, under the same climatic and geographical conditions as exist in this study area.
Materials and methods
Description of the study area
The Huize Pb–Zn Mining District (NE Yunnan Province, China; 26°63′ N, 103°71′ E), with total confirmed mineral deposits of more than 1.52 million tons and a vast resource area of approximately 74,000 ha, is located at the southwest end of the Yangzi Platform (Zhou et al., 2018). The research area (town of Kuangshan) is located in the northeastern portion of Huize County, which has a typical subtropical plateau monsoon climate with a mean annual temperature of 12.5°C, mean elevation of 2,200 m, mean annual sunlight of 2,100 h, and mean annual rainfall of 820 mm (data from China’s National Meteorological Information Center for the years 1990 to 2015). The natural soil type is brown or yellow-brown loam, and the natural vegetation is dominated by Pinus yunnanensis and Populus yunnanensis. The study area and sampling locations are shown in Figure 1 and Table 1.
Experimental design
This study was conducted in the summer of July to December 2021 at the Pb–Zn mining area and laboratory. Based on the locations of the major mine pits, we selected three sample regions (three replicates per sample region; Table 1) for collecting data. The three sample regions were all located on naturally restored mining terraces and separated from each other by more than 500 m. In each replicate, we chose C. nepalensis as the central point and established a 3 m × 3 m quadrat (CN quadrat) around it. Because of the large spatial heterogeneity of plant survival in the study area, four additional 3 m × 3 m quadrats (Non-CN quadrats) were established in all four major cardinal directions as control quadrats (all at a distance of 1 m from the CN quadrat) to represent the real situation as much as possible (Nyenda et al., 2020). In each plot, three plants were harvested to determine the related plant indicators (Functional traits and Biomass). Soil collection spots were arranged in all four major directions of the CN quadrat and at the central location of each Non-CN quadrat. The detailed layout of the sample points is shown in Figure 1.
Plant sampling and handling
We established a total of 45 quadrats for plant sample collection. In each quadrat, whole plants of selected individuals were harvested, shaken, placed in envelopes, and labeled. A metal frame with dimensions of 1 m × 1 m with 100 equally distributed grids (10 cm × 10 cm) was constructed above the canopy in each quadrat during cover measurement (Wang et al., 2018). We ensured that all plants collected had healthy root systems and no leaf chlorosis. The whole plants were carefully washed three times with tap water, rinsed with deionized water, and then height and root length were measured. Next, the samples were dried at 105°C for 30 min, then at 65°C for 48 h, and weighed to determine their biomass. A list of the native plants harvested is provided in Table 2.
Diversity index calculation
Complex biomass (CB), plant species richness (Simpson index, D, and Shannon–Wiener index, H), and Pielou evenness (J) indices were measured. The calculation methods are as follows:
Simpson index , (1) (Tang et al., 2020)
Shannon-Wiener index , (2) (Tang et al., 2020)
Pielou evenness index , (3) (Tang et al., 2020)
Complex biomass , (4) (Tang et al., 2020)
where S is the number of species in a quadrat, N is total number of individuals of each species in a quadrat, Pi is the ratio of the number of species i to the total number of individuals in a quadrat, and X is average biomass per species in a quadrat.
Five key survival functional traits (plant height, root length, cover, life form, and root system) known to be associated with the settlement of extreme habitats were selected for analysis. After dividing the functional traits into 11 categories, we computed multi-trait functional indices, including the functional richness index (FRic), functional evenness index (FEve), functional divergence index (FDiv), functional dispersion index (FDis), and Rao’s quadratic entropy index (RaoQ) using the “FD” package in R (Laliberté and Legendre, 2010). FRic is the area of functional trait space occupied by the organism in a community. FEve reflects the uniformity of distribution of functional traits in the ecological space within the community. FDiv indicates the difference in characteristic values of organisms in a community. FDis describes the mean distance in functional trait space for each species from the centroid of all species in an assemblage and reflects niche differentiation and resource competition of organisms within the community. RaoQ integrates the information of species richness and functional characteristics differences between species pairs and mainly calculates the variation in distance between species (Laliberté and Legendre, 2010).
Soil sampling and determination
We collected 72 soil samples (0–30 cm), which were analyzed for soil bulk density (SBD), pH, soil organic matter content (SOM), total nitrogen content (TN), total phosphorus content (TP), and soil heavy metal content (SHM). After removing stones and plant roots, the soil samples were air dried, ground to a fine powder, and then passed separately through 2 mm, 1 mm, and 0.25 mm sieves. SBD was measured using soil cores (volume of 100 cm3) and the volume ring technique (Li et al., 2016). Soil pH was determined using a water–soil ratio of 5:1 in an aqueous suspension. SOM was analyzed by the oxidation method, using K2Cr2O7–H2SO4 followed by titration with FeSO4 (Wu et al., 2015). Soil TN was analyzed using a Foss Kjeltec analyzer and the acid digestion method, respectively (Hu et al., 2018). To determine SHM, soil samples (0.5 g) were digested in HNO3:HClO4 (5:1, v/v) at 240°C for 4 h, filtered and diluted into 50 mL volumetric flasks with distilled water, and then the contents of Mn, Cu, Zn, Cd, and Pb in the extracts were measured via flame atomic absorption spectrophotometer (Agilent, 240 FS).
Quality assurance and statistical analyses
All indicator analyses were performed in triplicate, and the analytical values were calibrated using blank controls. The relative standard deviation of the three replicate measurements, except for blanks, was fixed at no more than 5%. Glassware was soaked in HCl (10% v/v) for 24 h and then rinsed with deionized water. Two continuous calibration standards (CCV) were tested for every 10 samples (5% of samples), and the calibration curve was linear with a regression coefficient (r)2 > 0.990. More importantly, quality assurance and quality control procedures were performed using the reference material GBW07405 (soil) received from the National Research Center for Reference Materials (Beijing, China).
Because of the imbalance between the datasets of plant communities of Non-CN and CN, new data were generated using the SMOTE algorithm to obtain balance (Chawla et al., 2002; Hong et al., 2021). The SMOTE algorithm was executed in R software with the “UBL” package (Branco et al., 2016). One-way analysis of variance (ANOVA) was used to assess the effects of the presence or absence of C. nepalensis on soil properties and plant complex characteristics. The Pearson correlation approach was utilized to examine the relationships between soil and plant complexes among the CN and Non-CN quadrats. A structural equation model (SEM) was constructed using the lavaan package in R (Rosseel, 2012), and the model parameter estimation method was based on the maximum likelihood method. The SEM was established to estimate the contribution rate and path of the primary influencing factor in the presence of C. nepalensis. All of the above statistical tests and illustrations drawn were executed in R version 4.1.3 or 4.2.0 (R Core Team, 2018).
Results
Effects of Coriaria nepalensis on soil properties and heavy metal contents
Soil TN (F = 20.706, p < 0.001), TP (F = 27.887, p < 0.001), and SOM (F = 141.881, p < 0.001) were significantly promoted in each sample region when C. nepalensis was present (Figure 2 and Table 3). In addition, the concentrations of Mn (F = 22.616, p < 0.001), Cu (F = 36.804, p < 0.001), Cd (F = 29.686, p < 0.001), Zn (F = 31.650, p < 0.001), and Pb (F = 50.401, p < 0.001) in soil were higher in the Non-CN quadrats than in the CN quadrats (Figure 3 and Table 3). The presence or absence of C. nepalensis did not affect SBD (F = 1.399, p < 0.001) or pH (F = 0.134, p < 0.001) across the sample regions (Figure 2 and Table 3). In summary, the main functions of C. nepalensis in the mining areas were to enhance soil fertility and reduce the contents of heavy metals in soil without changing the soil’s physical structure or acidity and alkalinity.
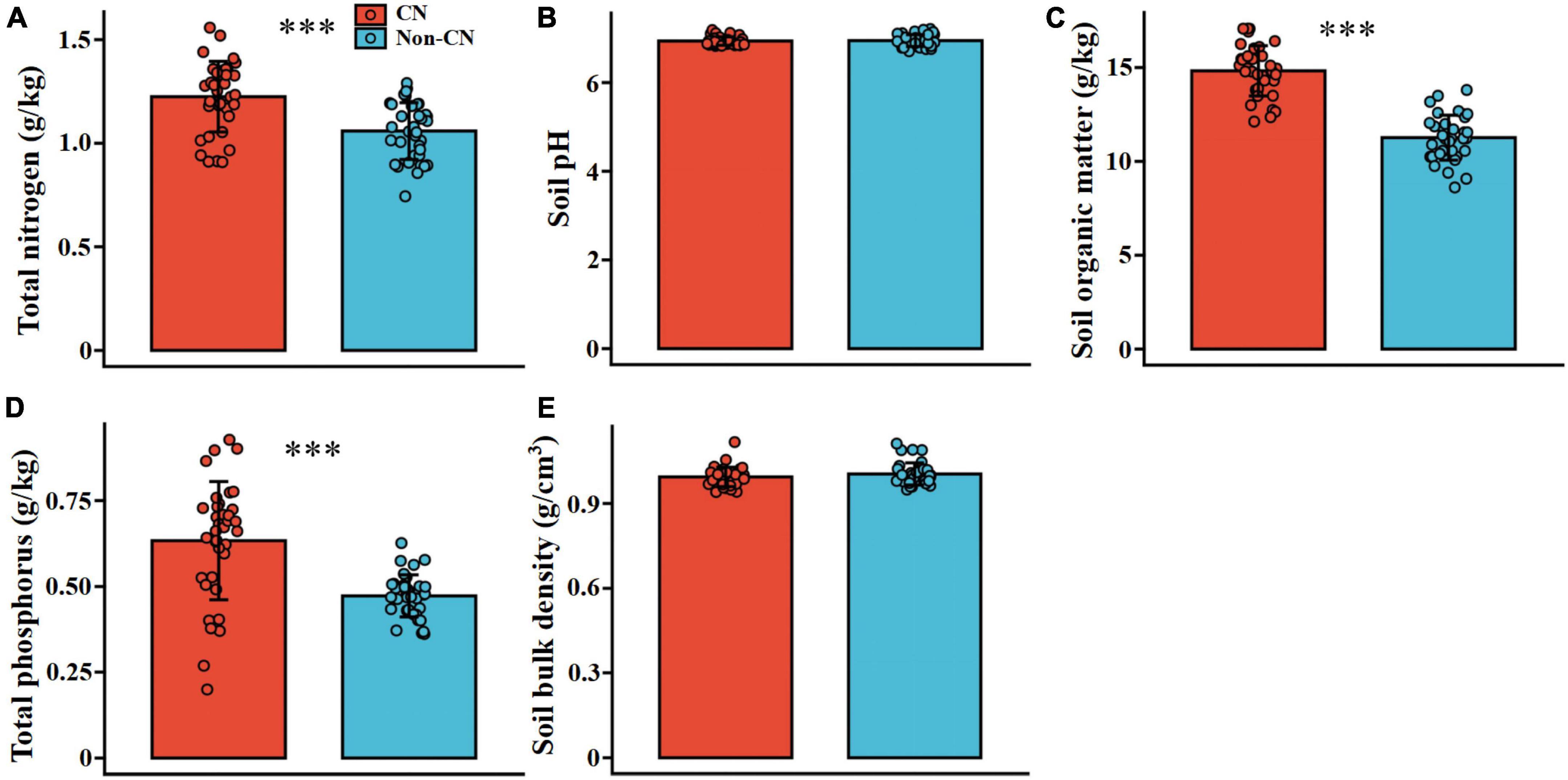
Figure 2. Total nitrogen (A), soil pH (B), soil organic matter (C), total phosphorus (D), and soil bulk density (E) in the presence (CN) or absence (Non-CN) of C. nepalensis in sample regions (n = 36). *** (p ≤ 0.001) indicate significant differences between CN and Non-CN.
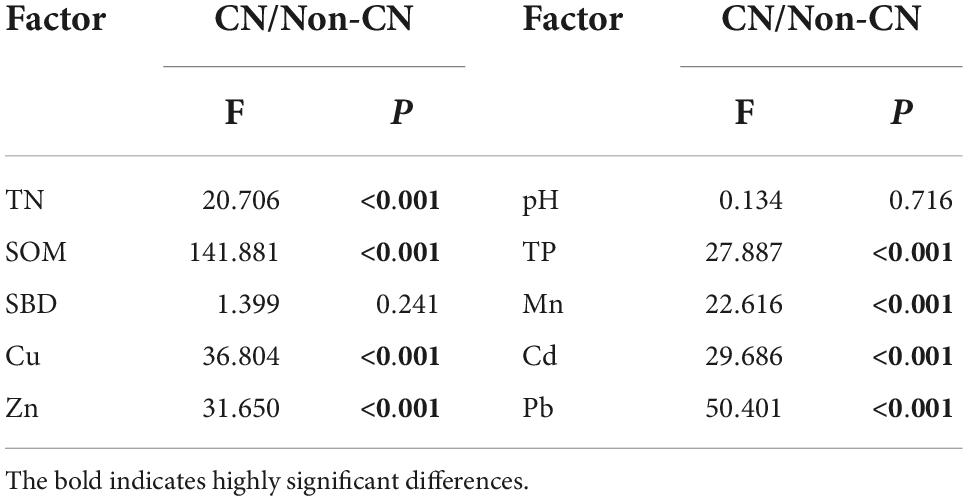
Table 3. Effects of presence or absence of C. nepalensis (CN/Non-CN) on total nitrogen (TN), soil pH (pH), soil organic matter (SOM), total phosphorus (TP), soil bulk density (SBD), and soil heavy metal contents (Mn, Cu, Cd, Zn, and Pb).
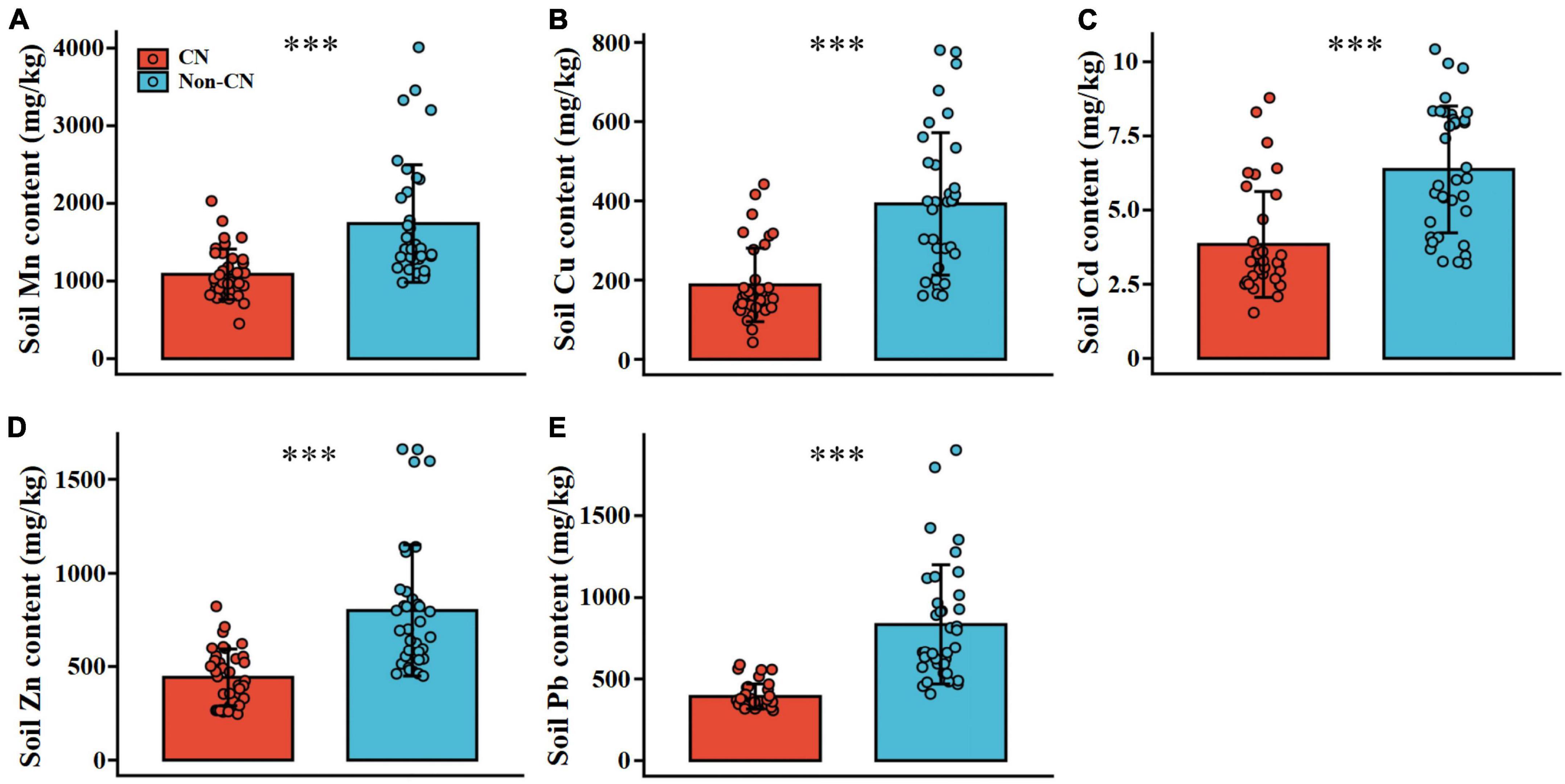
Figure 3. Soil contents of Mn (A), Cu (B), Cd (C), Zn (D), and Pb (E) in the presence (CN) or absence (Non-CN) of C. nepalensis in sample regions (n = 36). *** (p ≤ 0.001) indicate significant differences between CN and Non-CN.
Effects of Coriaria nepalensis on diversity, function, and biomass of plant complexes
CB (F = 13.642, p = 0.002), D (F = 32.210, p < 0.001), H (F = 31.967, p < 0.001), FDis (F = 13.564, p = 0.002), and RaoQ (F = 16.833, p = 0.001) were remarkably larger in the CN quadrats than the Non-CN quadrats (Figure 4 and Table 4). There were no significant differences related to the presence or absence of C. nepalensis for J (F = 3.420, p = 0.083), FRic (F = 1.086, p = 0.313), FEve (F = 1.114, p = 0.307), and FDiv (F = 2.176, p = 0.160) (Figure 4 and Table 4). The emergence of C. nepalensis changed the relationships among species, provided more living space for plants in different niches, increased species diversity, and increased functional diversity and community productivity, thus enhancing the stability of the mining ecosystem.
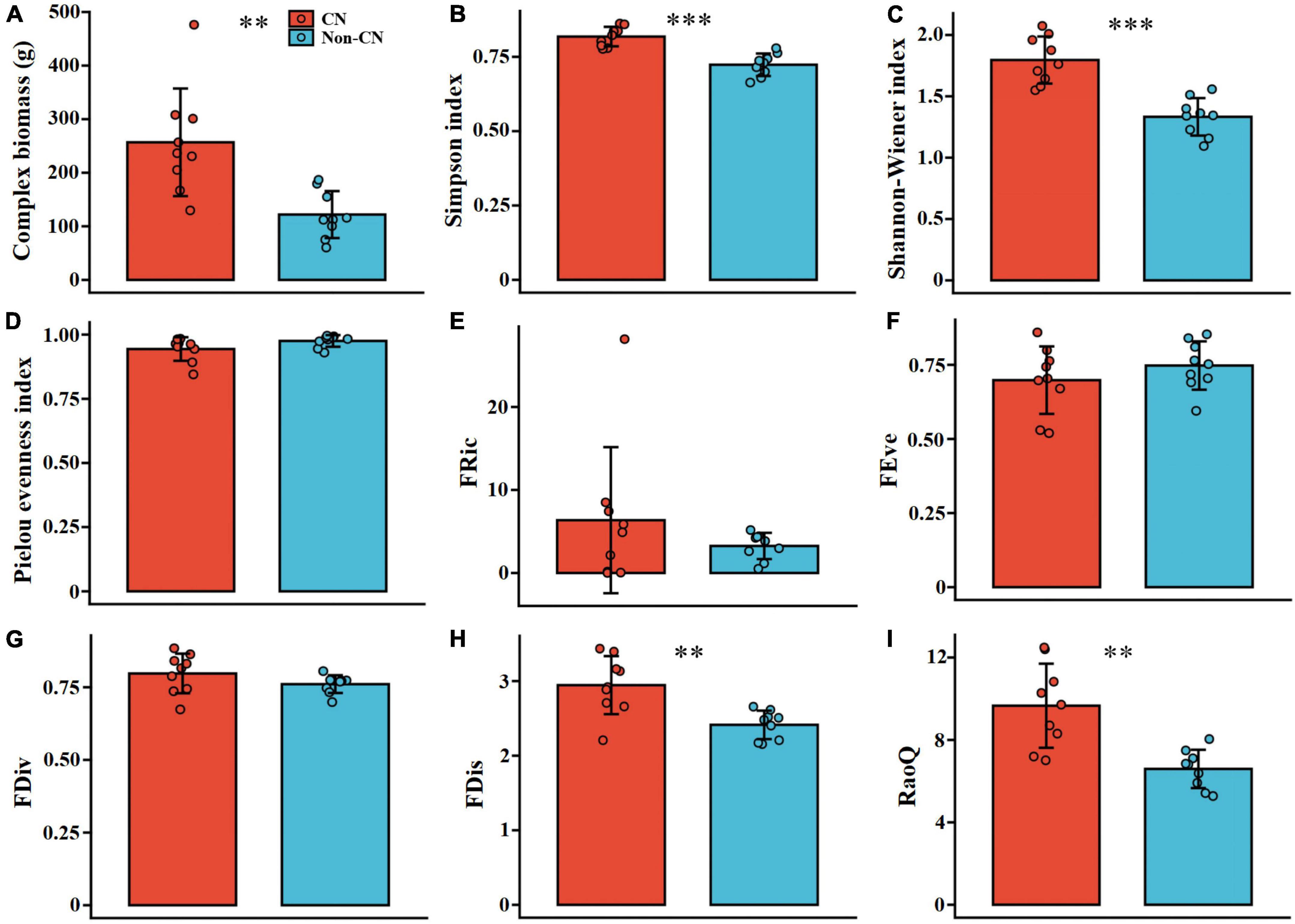
Figure 4. Complex biomass (A), Simpson index (B), Shannon–Wiener index (C), Pielou evenness index (D), functional richness index (E), functional evenness index (F), functional divergence index (G), functional dispersion index (H), and Rao’s quadratic entropy index (I) in the presence (CN) or absence (Non-CN) of C. nepalensis in sample regions (n = 9). Asterisks indicate significant differences between CN and Non-CN: ** (0.001 < p ≤ 0.01), *** (p ≤ 0.001).
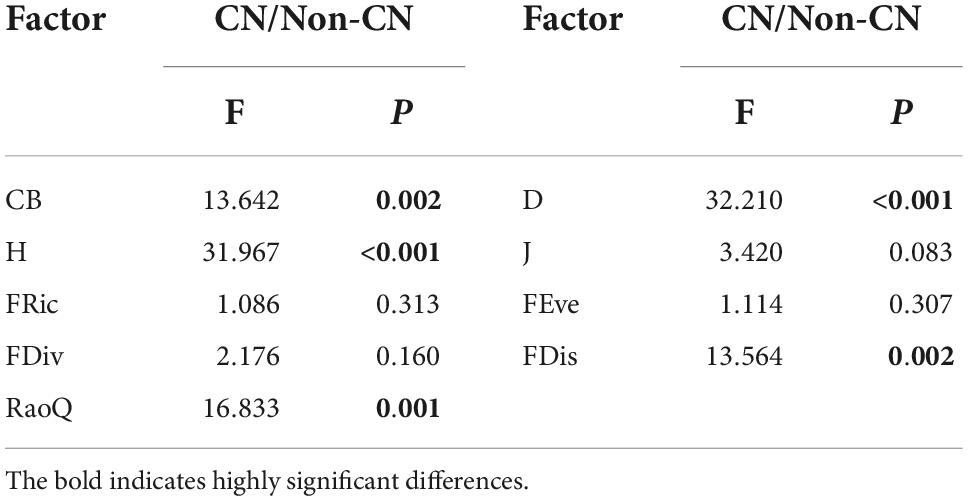
Table 4. Effects of presence or absence of C. nepalensis (CN/Non-CN) on complex biomass (CB), Simpson index (D), Shannon–Wiener index (H), Pielou evenness index (J), functional richness index (FRic), functional evenness index (FEve), functional divergence index (FDiv), functional dispersion index (FDis), and Rao’s quadratic entropy index (RaoQ).
Relationships among soil and plant complex characteristics in the presence and absence of Coriaria nepalensis
This research focused on the relationships between indicators with a correlation coefficient greater than 0.3 (Figure 5). In the CN quadrats, TN was positively related to H, FRic, FEve, and CB. H and CB entirely had a positive correlation with FRic and FEve, respectively. FDis was positively associated with Zn, CB, and FEve. SBD was positively and strongly related to Mn, Cu, and Cd. Mn, Cu, Cd, Zn, and Pb were positively associated with each other. In the Non-CN quadrats, D and H were negatively and strongly related to Pb, Cu, and Cd, and there were positive correlations among FRic, FEve, FDiv, FDis, and RaoQ. Strong positive correlations were observed among the Pb, Zn, Cu, and Mn contents in the soil. In addition, Pb, Zn, and Mn were positively associated with SBD. Therefore, in the study area, the presence of C. nepalensis allowed for better contact between the plant complex and the soil, increased the nitrogen content in the soil, and weakened the toxic effects of heavy metals on plants.
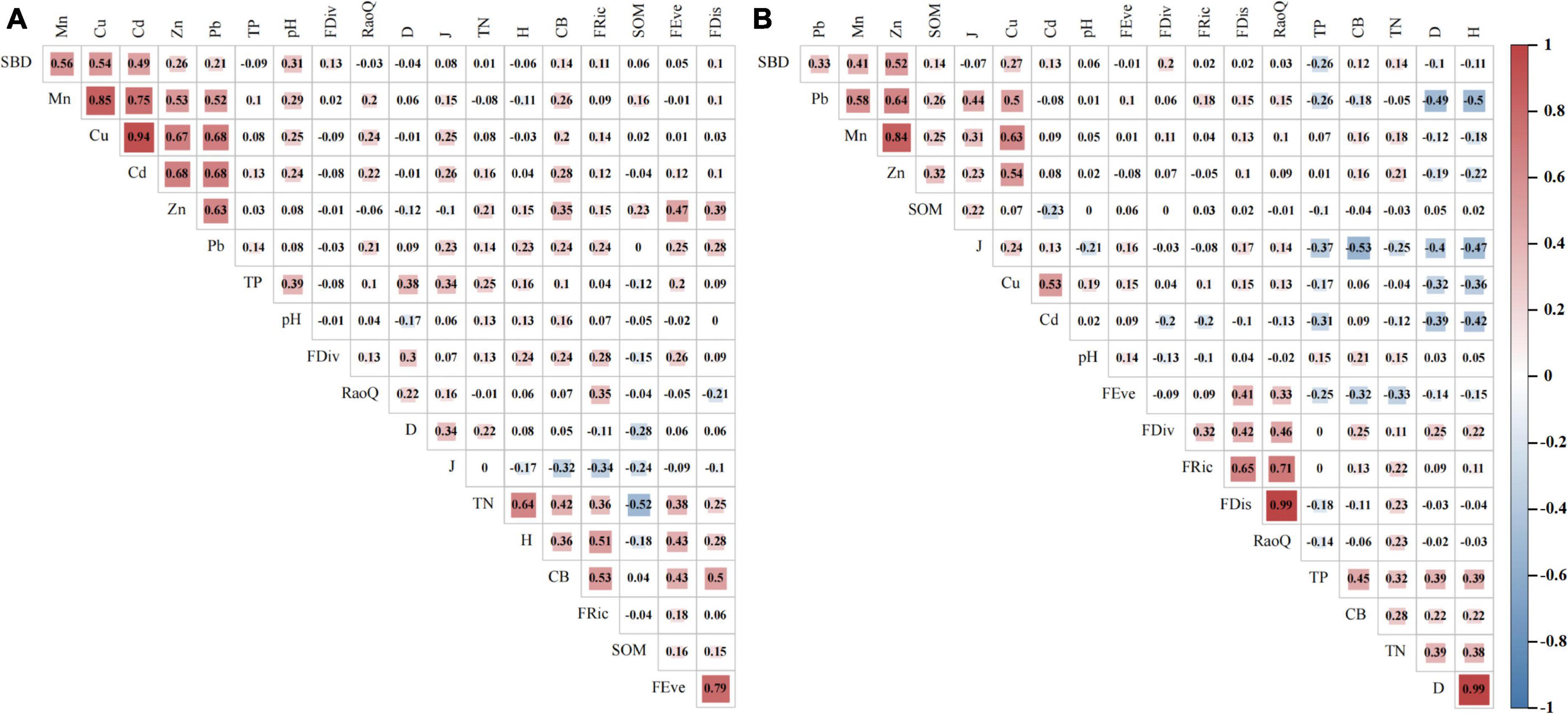
Figure 5. Pearson correlation coefficients of total nitrogen (TN), total phosphorus (TP), soil organic matter (SOM), soil bulk density (SBD), pH, soil heavy metal contents (Mn, Cu, Zn, Cd, and Pb), complex biomass (CB), Simpson index (D), Shannon–Wiener index (H), Pielou evenness index (J), functional richness index (FRic), functional evenness index (FEve), functional divergence index (FDiv), functional dispersion index (FDis), and Rao’s quadratic entropy index (RaoQ) in sample regions. (A) Indicates presence of C. nepalensis (CN), and (B) Indicates absence of C. nepalensis (Non-CN).
Structural equation model of Coriaria nepalensis stabilization of plant complexes
According to Figure 5A, the presence of C. nepalensis changed the plant–soil feedback, which might directly or indirectly affect complex biomass. Therefore, SEM was used to explore the key factors affecting complex biomass (Figure 6). The Non-CN quadrats were used as controls. In the CN quadrats, the effects could be divided into three main paths: (i) FDis → CB (λ = 0.47), (ii) FRic → CB (λ = 0.52), and (iii) TN → H (λ = 0.64) → FRic (λ = 0.54) → CB (λ = 0.52). In the Non-CN quadrats, there were two major paths: (i) TN → H (λ = 0.38) and (ii) FDis → FRic (λ = 0.66). When C. nepalensis was present, FRic and FDis were direct factors affecting the biomass of the community, while TN and H were indirect factors. When C. nepalensis was not present, the soil nitrogen content affected plant richness, and the differences in plant characters was the factor maintaining the functional richness. Overall, nitrogen may be the main limiting factor of plant colonization and survival in the mining ecosystem.
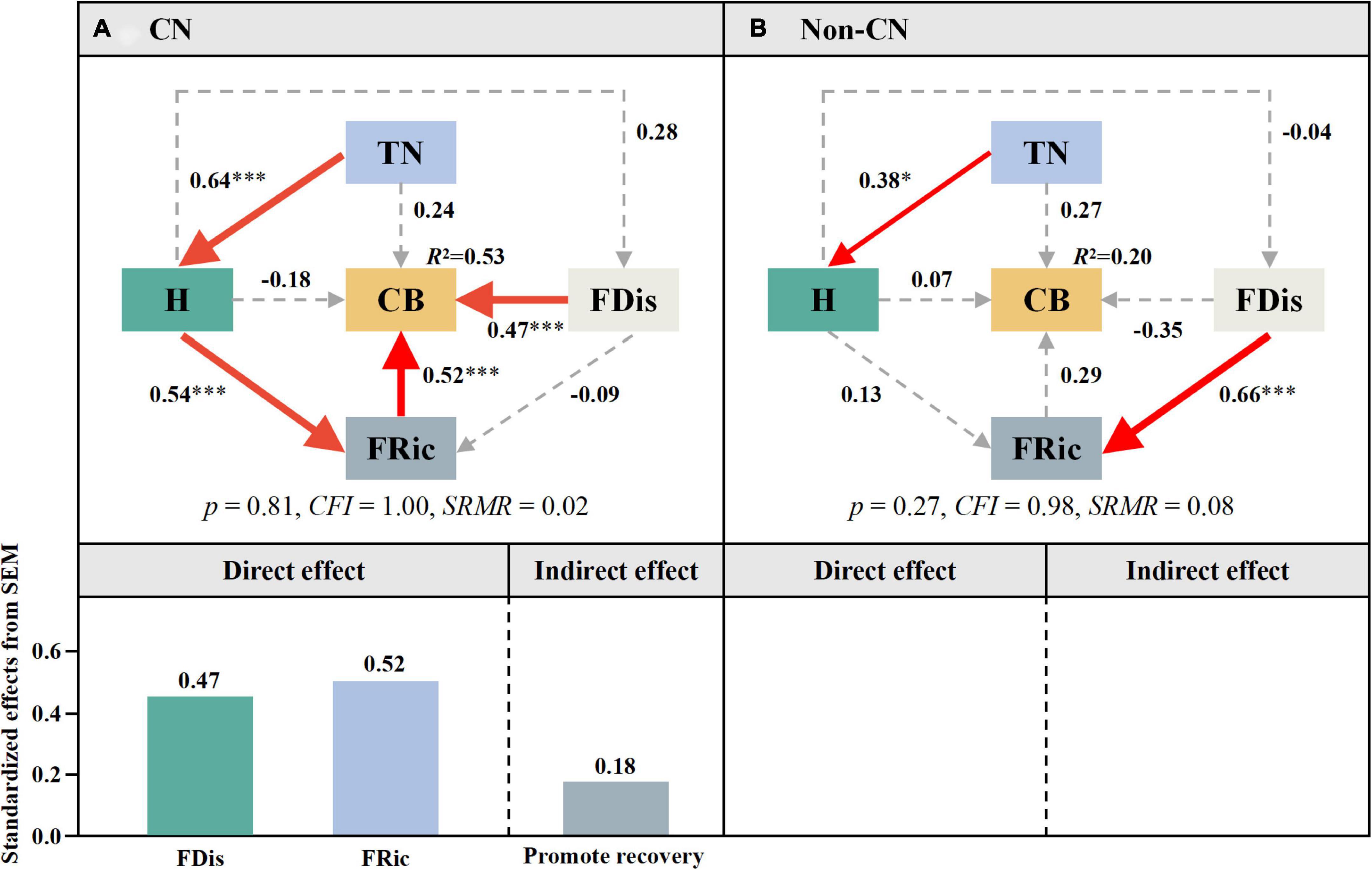
Figure 6. Effects of C. nepalensis from SEM. Total nitrogen content (TN), complex biomass (CB), Shannon–Wiener index (H), functional richness index (FRic), and functional dispersion index (FDis). Path coefficients are adjacent to arrows. Red lines indicate significant positive pathways. Asterisks indicate significant differences between CN (A) and Non-CN (B): * (0.01 < p < 0.05), *** (p ≤ 0.001).
Discussion
Influence of Coriaria nepalensis on soil properties
Our findings indicate that there was large spatial heterogeneity of relevant soil indicators in the study area, which was due to the different timings, methods, and intensities of mining (Shi et al., 2022). To verify the role of C. nepalensis in the restoration process of abandoned Pb–Zn mining areas, we tested whether it could positively modify soil properties. When C. nepalensis was present, soil TN, TP, and organic matter increased significantly, while SHM contents decreased dramatically (Figures 2, 3 and Table 3). C. nepalensis is a non-legume actinorhizal N-fixing species with root nodules (Yan et al., 2017), and the arbuscular mycorrhizal fungi in concert with nodulating bacteria in its roots effectively fix nitrogen from the soil and air (Tiwari et al., 2003; Manral et al., 2022). Fang et al. (2008) and Awasthi et al. (2022c) proved that C. nepalensis was more important as a nitrogen source than grass or ferns in studies of artificial forests and natural forests on upland sites in Southwest China and Northeast India. This is the reason for the significant increase in nitrogen in the microenvironment around C. nepalensis. The accumulation, decay, and decomposition of litter are the main reasons for the increase in soil organic matter. Using 15N isotope tracing, Wang et al. (2021) found that an increase in nitrogen significantly enhanced ecosystem function in a subtropical forest in China. Mineral-bound phosphorus is the main form of phosphorus present in nature. However, nitrogen enrichment can promote the dissolution of recalcitrant mineral-bound phosphorus into available phosphorus, thus increasing the phosphorus content of the soil (Wang et al., 2022). Cao et al. (2017) dried, ground, sieved, distilled, and filtered C. nepalensis plant material to produce plant washing agents and suggested that they had excellent removal effects for Pb, Zn, and Cd in soil. Therefore, C. nepalensis can secrete substances capable of removing heavy metal elements, reducing the contents of heavy metals around its roots.
The presence of C. nepalensis did not change the acidity and alkalinity of the soil but did increase the correlation between heavy metal elements and SBD (Figure 5). The development of vegetation in mining areas alters the soil porosity, which is an important driving factor for strengthening the relationship with heavy metals (Ciarkowska, 2017). The main reasons for the change in the relationship with heavy metal elements are that an increase in nutrient elements can promote the accumulation of heavy metal elements in plants, nutrient elements can form insoluble salts that directly absorb surface heavy metal ions or induce and enhance their adsorption by soil, and nutrient element ions can precipitate with heavy metal ions (Baragano et al., 2021). C. nepalensis roots may secrete a variety of cell-active substances, which may regulate soil nutrient elements and heavy metal elements. More attention should be given to this topic in future research.
Effect of Coriaria nepalensis on plant complexes
Our research showed that the presence of C. nepalensis facilitated the maintenance of more species and increased high-level niche differentiation, resulting in larger complex biomass (Figure 4 and Table 4), which is consistent with research on phosphorus-rich soil (Yan et al., 2017) and hillsides with serious surface erosion. C. nepalensis had a strong promoting effect in the harsh environment, in which the α-diversity and biomass of herbs growing under the canopy of C. nepalensis were significantly higher than those of herbs growing in open air (Joshi et al., 2001). Moreover, in the highly degraded forests in the Indian central Himalaya, some research (Kumar and Ram, 2005; Mourya et al., 2019) has demonstrated that C. nepalensis helps restore original vegetation composition by triggering forest renewal.
Traditional ecological theory suggests that the abiotic component of the species niche is the sole determinant of species establishment and growth under extreme conditions. However, this view ignores the interactions between organisms that may realign the species’ fundamental niche, resulting in a final ecological niche filtered through abiotic and biotic effects (Navarro-Cano et al., 2018). The use of inter-plant facilitation to construct plant patches as a means of mine rehabilitation is an emerging direction of research in the field of ecology (Wang et al., 2014b). Field surveys performed to select nurse species that are resistant and can facilitate the colonization and survival of other plants are a critical step toward this restoration approach. Therefore, studying the effects of nurse plants on plant diversity, complex biomass, and soil properties is essential for mining area restoration.
Effect of Coriaria nepalensis on changing plant–soil feedbacks
Soil and plants, as the basic structural units of the ecosystem, play an important role in the ecological restoration of mining areas, and there are complicated interactions between them (Li et al., 2021). In this research, when C. nepalensis was not present, the strong toxicity of heavy metals led to lower plant diversity and more intense competition among species (Figure 5B). However, when C. nepalensis was present, the opposite occurred. TN was positively related to H, FRic, FEve, and CB (Figure 5A). According to structural equation modeling (Figure 6), the presence of C. nepalensis increased the nitrogen content in the soil, promoted increases in complex biomass and niche complementarity, and enhanced the adaptability of auxiliary species to heavy metal pollution. In abandoned Pb–Zn mining areas, the contents of nutrient elements in the soil matrix are a key factor that restricts plant colonization (Cross et al., 2021a). Cross et al. (2021b) suggested that the germination of seeds, survival of seedlings, and growth of plants are positively correlated with the nitrogen in the soil, which indicates that nitrogen (available N; Li and Liber, 2018) is the decisive factor limiting vegetation restoration in mining areas. Many ecological studies (Perroni-Ventura et al., 2010; Huang et al., 2012) have defined fertility islands as areas of trees or shrubs (usually nitrogen-fixing plants) under abiotic stress, and the areas beneath these canopies have higher concentrations of nitrogen and organic matter than areas outside of the canopies. Such fertility islands, also known as survival islands, can promote improvement of the local soil matrix and form stable vegetation patches (Perroni-Ventura et al., 2010). Compared to adjacent bare land, it can create better resource pools and physical and chemical properties in or near the rhizosphere (Nyenda et al., 2020). In summary, C. nepalensis is a nurse plant that can form fertility islands in extreme habitats.
Rich species and functional traits greatly promote the amount of community biomass and the stability of the ecosystem (Qiu and Cardinale, 2020). The diversity of plants depends on the feedbacks in the ecosystem and the complementarity among species, and these accumulate over time (Reich et al., 2012). The plant patches (survival islands) in the mining area provide a basis for a community of highly diverse species with redundant functions and the development of a more stable ecosystem over time. Furthermore, Risueno et al. (2020a) suggested that plant patches have a positive effect on soil microorganisms, stimulating them to enhance the biogeochemical cycle. Thus, C. nepalensis can greatly facilitate vegetation restoration and control of heavy metal pollution in mining areas.
Beyond discovering the functions and roles of C. nepalensis in difficult habitats, it is important to determine why C. nepalensis aids the settlement of other species and to define the relevant ecological principle, which we did not consider. Xu et al. (2020) identified and explained the formation mechanism of the relationship between leguminous plants and their neighbors in 11 large forest plots (16–60 ha) around the world. They pointed out that this relationship is an atypical “altruism” and that its essence is still “egoism.” In extreme cases, there is almost no competition for nitrogen resources between nitrogen-fixing plants and their neighbors. These plants pass through stages of first being “selfish,” then “beneficial to others,” and finally to a state of mutual benefit and harmonious coexistence.
We hope that future researchers give more attention to the roles of other nurse plants in abandoned mining area land when conducting field investigations. The best way to rehabilitate is to adapt to the local conditions and allow nature to develop.
Conclusion
In the course of natural succession of abandoned land in the mining area, nurse plants contribute to the formation of fertility islands (survival island), which is beneficial for other plants to colonize, survive and grow. This is essential for promoting community reconstruction, as confirmed by this study on the positive impact of Coriaria nepalensis as a nurse plant on soil properties, such as increasing the content of nutrient elements and reducing the content of heavy metals in the soil, and on plant patch formation, such as increasing the patch biomass and reducing the intensity of interspecific competition. Therefore, it is suggested that future research can be focused on exploring the law of natural succession followed by the nurse plants in mining areas.
Data availability statement
The original contributions presented in this study are included in the article/supplementary material, further inquiries can be directed to the corresponding author.
Author contributions
X-QY: conceptualization, methodology, software, writing – original draft, visualization, and investigation. Z-LG: investigation and software. C-QD: methodology, validation, revision, review and editing, supervision, and funding acquisition. JY, HT, and L-YL: investigation. TL and C-EL: methodology. All authors contributed to the article and approved the submitted version.
Funding
This research was supported by the National Natural Science Foundation of China (U2002208 and 32260315) and China Yunnan Provincial R&D Programs (202101AS070033, 202201AS070016, and 202201BF070001-002).
Acknowledgments
We are grateful for the experimental site provided by Yunnan University and the staff in the mining area of the Kuangshan town.
Conflict of interest
The authors declare that the research was conducted in the absence of any commercial or financial relationships that could be construed as a potential conflict of interest.
Publisher’s note
All claims expressed in this article are solely those of the authors and do not necessarily represent those of their affiliated organizations, or those of the publisher, the editors and the reviewers. Any product that may be evaluated in this article, or claim that may be made by its manufacturer, is not guaranteed or endorsed by the publisher.
References
Awasthi, P., Bargali, K., Bargali, S. S., and Jhariya, M. K. (2022a). Structure and functioning of Coriaria nepalensis wall dominated shrublands in degraded hills of Kumaun Himalaya. I. dry matter dynamics. Land Degrad Dev 33, 1474–1494. doi: 10.1002/ldr.4235
Awasthi, P., Bargali, K., Bargali, S. S., Khatri, K., and Jhariya, M. K. (2022b). Nutrient partitioning and dynamics in Coriaria nepalensis wall dominated shrublands of degraded hills of Kumaun Himalaya. Front. For. Glob. Change 5:913127. doi: 10.3389/ffgc.2022.913127
Awasthi, P., Bargali, K., and Bargali, S. S. (2022c). Relative performance of woody vegetation in response to facilitation by Coriaria nepalensis in Central Himalaya, India. Russ. J. Ecol. 53, 191–203. doi: 10.1134/S1067413622030031
Baragano, D., Gallego, J. L. R., and Forjan, R. (2021). Short-term experiment for the in situ stabilization of a polluted soil using mining and biomass waste. J. Environ. Manage. 296:113179. doi: 10.1016/j.jenvman.2021.113179
Bateman, A. M., Erickson, T. E., Merritt, D. J., Veneklaas, E. J., and Muñoz-Rojas, M. (2021). Native plant diversity is a stronger driver for soil quality than inorganic amendments in semi-arid post-mining rehabilitation. Geoderma 394:115001. doi: 10.1016/j.geoderma.2021.115001
Bolan, N. S., Park, J. H., Robinson, B., Naidu, R., and Huh, K. Y. (2011). Phytostabilization: a green approach to contaminant containment. Adv. Agron. 112, 145–204. doi: 10.1016/B978-0-12-385538-1.00004-4
Branco, P., Ribeiro, R. P., and Torgo, L. (2016). UBL: an R package for utility-based learning. arXiv [Preprint]. doi: 10.48550/arXiv.1604.08079
Cao, Y. R., Zhang, S. R., Wang, G. Y., Huang, Q. L., Li, T., and Xu, X. X. (2017). Removal of Pb, Zn, and Cd from contaminated soil by new washing agent from plant material. Environ. Sci. Pollut. Res. 24, 8525–8533. doi: 10.1007/s11356-017-8542-3
Chawla, N. V., Bowyer, K. W., Hall, L. O., and Kegelmeyer, W. P. (2002). SMOTE: synthetic minority over-sampling technique. J. Artif. Intell. Res. 16, 321–357. doi: 10.1613/jair.953
Ciarkowska, K. (2017). Organic matter transformation and porosity development in non-reclaimed mining soils of different ages and vegetation covers: a field study of soils of the zinc and lead ore area in SE Poland. J. Soils Sediments 17, 2066–2079. doi: 10.1007/s11368-017-1678-4
Coelho, P. C. S., Teixeira, J. P. F., and Gonçalves, O. N. B. S. M. (2011). “Mining activities: health impacts,” in Encyclopedia of Environmental Health, Vol. 3, ed. J. O. Nriagu (Burlington: Elsevier), 788–802.
Cross, A. T., Stevens, J. C., Sadler, R., Moreira-Grez, B., Ivanov, D., Zhong, H. T., et al. (2021a). Compromised root development constrains the establishment potential of native plants in unamended alkaline post-mining substrates. Plant Soil 461, 163–179. doi: 10.1007/s11104-018-3876-2
Cross, A. T., Ivanov, D., Stevens, J. C., Sadler, R., Zhong, H. T., Lambers, H., et al. (2021b). Nitrogen limitation and calcifuge plant strategies constrain the establishment of native vegetation on magnetite mine tailings. Plant Soil 461, 181–201. doi: 10.1007/s11104-019-04021-0
Fang, S. Z., Li, H. Y., and Xie, B. D. (2008). Decomposition and nutrient release of four potential mulching materials for poplar plantations on upland sites. Agroforest. Syst. 74, 27–35. doi: 10.1007/s10457-008-9155-0
Festin, S. E., Tigabu, M., Chileshe, N. M., Syampungani, S., and Oden, P. C. (2019). Progresses in the restoration of the post-mined landscape in Africa. J. For. Res. 30, 381–396. doi: 10.1007/s11676-018-0621-x
Gosain, B. G., Negi, G. C. S., Dhyani, P. P., Bargali, S. S., and Saxena, R. (2015). Ecosystem services of forests: carbon Stock in vegetation and soil components in a watershed of Kumaun Himalaya, India. Int. J. Ecol. Environ. Sci. 41, 177–188.
Haddaway, N. R., Cooke, S. J., Lesser, P., Macura, B., Nilsson, N. E., Taylor, J. J., et al. (2019). Evidence of the impact of mental mining and the effectiveness of mining mitigation measures on social-ecological systems in Artic and Arboreal regions; a systematic map protocol. Environ. Evid. 8:9. doi: 10.1186/s13750-022-00282-y
Hong, Y. S., Chen, Y. Y., Shen, R. L., Chen, S. C., Xu, G., Cheng, H., et al. (2021). Diagnosis of cadmium contamination in urban and suburban soils using visible-to-near-infrared spectroscopy. Environ. Pollut. 291:118128. doi: 10.1016/j.envpol.2021.118128
Hooper, D. U., Chapin, F. S., Ewel, J. J., Hector, A., Inchausti, P., Lavorel, S., et al. (2005). Effects of biodiversity on ecosystem functioning: a consensus of current knowledge. Ecol. Monogr. 75, 3–35. doi: 10.1890/04-0922
Hossain, D., Gorman, D., Chapelle, B., Mann, W., Saal, R., and Penton, G. (2013). Impact of the mining industry on the mental health of landholders and rural complexesin southwest Queensland. Australas. Psychiatr. 2013, 32–37. doi: 10.1177/1039856212460287
Hu, Y. L., Mgelwa, A. S., Singh, A. N., and Zeng, D. H. (2018). Differential responses of the soil nutrient status, biomass production, and nutrient uptake for three plant species to organic amendments of placer gold mine–tailing soils. Land Degrad. Dev. 29, 2836–2845. doi: 10.1002/ldr.3002
Huang, L., Baumgartl, T., and Mulligan, D. (2012). Is rhizosphere remediation sufficient for sustainable revegetation of mine tailings? Ann. Bot. 110, 223–238. doi: 10.1093/aob/mcs115
Ilunga, E. I. W., Mahy, G., Piqueray, J., Séleck, M., Shutcha, M. N., Meerts, P., et al. (2015). Plant functional traits as a promising tool for the ecological restoration of degraded tropical metal-rich habitats and revegetation of metal-rich bare soils: a case study in copper vegetation of Katanga, DRC. Ecol. Eng. 82, 214–221. doi: 10.1016/j.ecoleng.2015.04.084
Jia, P., Liang, J. L., Yang, S. X., Zhang, S. C., Liu, J., Liang, Z. W., et al. (2020). Plant diversity enhances the reclamation of degraded lands by stimulating plant-soil feedbacks. J. Appl. Ecol. 57, 1258–1270. doi: 10.1111/1365-2664.13625
Joshi, B., Singh, S. P., Rawat, Y. S., and Goel, D. (2001). Facilitative effect of Coriaria nepalensis nepalensis on species diversity and growth of herbs on severely eroded hill slopes. Curr. Sci. 80, 678–682.
Köbel, M., Listopad, C. M. C. S., Príncipe, A., Nunes, A., and Branquinho, C. (2021). Temporary grazing exclusion as a passive restoration strategy in a dryland woodland: effects over time on tree regeneration and on the shrub complex. For. Ecol. Manag. 483:118732. doi: 10.1016/j.foreco.2020.118732
Kumar, A., and Ram, J. (2005). Anthropogenic disturbances and plant biodiversity in forests of Uttaranchal, central Himalaya. Biodivers. Conserv. 14, 309–331. doi: 10.1007/s10531-004-5047-4
Laliberté, E., and Legendre, P. (2010). A distance-based framework for measuring functional diversity from multiple traits. Ecology 91, 299–305. doi: 10.1890/08-2244.1
Lei, D. M., and Duan, C. Q. (2008). Restoration potential of pioneer plants growing on lead-zinc mine tailings in Lanping, southwest China. J. Environ. Sci. 20, 1202–1209. doi: 10.1016/S1001-0742(08)62210-X
Li, S., and Huang, L. (2015). Toward a new paradigm for tailings phytostabilization-nature of the substrates, amendment options, and anthropogenic pedogenesis. Crit. Rev. Environ. Sci. Technol. 45, 813–839. doi: 10.1080/10643389.2014.921977
Li, S. Q., and Liber, K. (2018). Influence of different revegetation choices on plant complex and soil development nine years after initial planting on a reclaimed coal gob pile in the Shanxi mining area, China. Sci. Total Environ. 618, 1314–1323. doi: 10.1016/j.scitotenv.2017.09.252
Li, T., Wu, M. H., Duan, C. Q., Li, S. H., and Liu, C. E. (2022). The effect of different restoration approaches on vegetation development in metal mines. Sci. Total Environ. 806:159626. doi: 10.1016/j.scitotenv.2021.150626
Li, W. Z., Wang, J. M., Zhang, Y. F., and Zhang, M. (2021). A novel characterization on the interaction of soil and vegetation in a reclaimed area of opencast coalmine based on joint multi-fractal method. Ecol. Indic. 121:107094. doi: 10.1016/j.ecolind.2020.107094
Li, Y., Gao, Z., and Tang, L. (2016). Soil-plant characteristics in an age sequence of Coronilla varia L. plantations along embankments. J. Soil Sci. Plant Nutr. 16, 187–199. doi: 10.4067/S0718-95162016005000015
Li, Z. R., Colinet, G., Zu, Y. Q., Wang, J. X., An, L. Z., Li, Q., et al. (2019). Species diversity of Arabis alpina L. communities in two Pb/Zn mining areas with different smelting history in Yunnan Province, China. Chemosphere 233, 603–614. doi: 10.1016/j.chemosphere.2019.05.228
Liu, L. W., Li, W., Song, W. P., and Guo, M. X. (2018). Remediation techniques for heavy metal-contaminated soils: principles and applicability. Sci. Total Environ. 633, 206–219. doi: 10.1016/j.scitotenv.2018.03.161
Liu, X. H., and Liu, S. L. (2020). Introduction to the special issue: biodiversity mechanism in natural succession and ecological restoration. Ecol. Eng. 143:105614. doi: 10.1016/j.ecoleng.2019.105614
Loreau, M., and de Mazancourt, C. (2013). Biodiversity and ecosystem stability: a synthesis of underlying mechanisms. Ecol. Lett. 16, 106–115. doi: 10.1111/ele.12073
Manral, V., Bargali, K., Bargali, S. S., Jhariya, M. K., and Padalia, K. (2022). Relationships between soil and microbial biomass properties and annual flux of nutrients in Central Himalayan forests, India. Land Degrad. Dev. 33, 2014–2025. doi: 10.1002/ldr.4283
Martínez-Fernández, D., Walker, D. J., Romero-Espinar, P., Flores, P., and delRío, J. A. (2011). Physiological responses of Bituminaria bituminosa to heavy metals. J. Plant Physiol. 168, 2206–2211. doi: 10.1016/j.jplph.2011.08.008
Mensah, A. K. (2015). Role of revegetation in restoring the fertility of degraded mined soils in Ghana: a review. Int. J. Biodivers. Conserv. 7, 57–80. doi: 10.5897/IJBC2014.0775
Mourya, N. R., Bargali, K., and Bargali, S. S. (2019). Impacts of Coriaria nepalensis colonization on vegetation structure and regeneration dynamics in a mixed conifer forest of Indian Central Himalaya. J. For. Res. 30, 305–317. doi: 10.1007/s11676-018-0613-x
Munford, K. E., Watmough, S. A., Rivest, M., Poulain, A., Basiliko, N., and Mykytczuk, N. C. S. (2020). Edaphic factors inflfluencing vegetation colonization and encroachment on arsenical gold mine tailings near Sudbury, Ontario. Environ. Pollut. 264:114680. doi: 10.1016/j.envpol.2020.114680
Navarro-Cano, J. A., Verdú, M., Goberna, M., and Goberna, M. (2018). Trait- based selection of nurse plants to restore ecosystem functions in mine tailings. J. Appl. Ecol. 55, 1195–1206. doi: 10.1111/1365-2664.13094
Nyenda, T., Gwenzi, W., Gwata, C., and Jacobs, S. M. (2020). Leguminous tree species create islands of fertility and influence the understory vegetation on nickel-mine tailings of different ages. Ecol. Eng. 155:10590. doi: 10.1016/j.ecoleng.2020.105902
Padilla, F. M., and Pugnaire, F. I. (2006). The role of nurse plants in the restoration of degraded environments. Front. Ecol. Environ. 4:196–202. doi: 10.1890/1540-92952006004[0196:TRONPI]2.0.CO;2
Pan, H. J., Zhou, G. H., Cheng, Z. Z., Yang, R., He, L., Zeng, D. M., et al. (2014). Advances in geochemical survey of mine tailings project in China. J. Geochem. Explor. 139, 193–200. doi: 10.1016/j.gexplo.2013.07.012
Parraga-Aguado, I., Gonzalez-Alcaraz, M. N., Alvarez-Rogel, J., Jimenez-Carceles, F. J., and Conesa, H. M. (2013). The importance of edaphic niches and pioneer plant species succession for the phytomanagement of mine tailings. Environ. Pollut. 176, 134–143. doi: 10.1016/j.envpol.2013.01.023
Perroni-Ventura, Y., Montana, C., and Garcia-Oliva, F. (2010). Carbon-nirogen interaction in fertility island soil from a tropical semi-arid ecosystem. Funct. Ecol. 24, 233–242. doi: 10.1111/j.1365-2435.2009.01610.x
Qiu, J. X., and Cardinale, B. J. (2020). Scaling up biodiversity-ecosystem function relationships across space and over time. Ecology 101:e03166. doi: 10.1002/ecy.3166
R Core Team (2018). R: A Language and Environment for Statistical Computing. Vienna: R Foundation for Statistical Computing.
Reich, P. B., Tilman, D., Isbell, F., Mueller, K., Hobbie, S. E., Flynn, D. F. B., et al. (2012). Impacts of biodiversity loss escalate through time as redundancy fades. Science 336, 589–592. doi: 10.1126/science.1217909
Risueno, Y., Petri, C., and Conesa, H. M. (2020b). Edaphic factors determining the colonization of semiarid mine tailings by a ruderal shrub and two tree plant species: implications for phytomanagement. Chemosphere 259:127425. doi: 10.1016/j.chemosphere.2020.127425
Risueno, Y., Petri, C., and Conesa, H. M. (2020a). The importance of edaphic niches functionality for the sustainability of phytomanagement in semiarid mining impacted ecosystems. J. Environ. Manage. 266:110613. doi: 10.1016/j.jenvman.2020.110613
Rosseel, Y. (2012). lavaan: an R package for structural equation modeling. J. Stat. Softw. 48, 1–36. doi: 10.18637/jss.v048.i02
Saikia, P., and Khan, M. L. (2012). Seedling survival and growth of Aquilaria malaccensis Lam. in different microclimatic conditions of northeast India. J. For. Res. 23, 569–574.
Seenivasan, R., Prasath, V., and Mohanraj, R. (2015). Restoration of sodic soils involving chemical and biological amendments and phytoremediation by Eucalyptus camaldulensis in a semiarid region. Environ. Geochem. Health 37, 575–586. doi: 10.1007/s10653-014-9674-8
Shi, J., Du, P., Luo, H. L., Wu, H., Zhang, Y. H., Chen, J., et al. (2022). Soil contamination with cadmium and potential risk around various mines in China during 2000-2020. J. Environ. Manage. 310:114509. doi: 10.1016/j.jenvman.2022.114509
Tang, C. Q., Han, P. B., Li, S. F., Shen, L. Q., Huang, D. S., Li, Y. F., et al. (2020). Species richness, forest types and regeneration of Schima in the subtropical forest ecosystem of Yunnan, Southwestern China. For. Ecosyst. 7:35. doi: 10.1186/s40663-020-00244-1
Teixeira, L. A. J., Berton, R. S., Coscione, A. R., and Saes, L. A. (2011). Biosolids application on banana production: soil chemical properties and plant nutrition. Appl. Environ. Soil Sci. 2011:238185. doi: 10.1155/2011/238185
Tiwari, M., Singh, S. P., Tiwari, A., and Sundriyal, R. C. (2003). Effect of symbiotic associations on growth of host Coriaria nepalensis and its facilitative impact on oak and pine seedlings in the Central Himalaya. For. Ecol. Manag. 184, 141–147. doi: 10.1016/S0378-1127(03)00209-3
Wang, A., Chen, D. X., Phillips, O. L., Gundersen, P., Zhou, X. L., and Gurmesa, G. A. (2021). Dynamics and multi-annual fate of atmospherically deposited nitrogen in montane tropical forests. Glob. Chang. Biol. 27, 2076–2087. doi: 10.1111/gcb.15526
Wang, J., Ge, Y., Chen, T., Bai, Y., Quian, B. Y., and Zhang, C. B. (2014b). Facilitation drives the positive effects of plant richness on trace metal removal in a biodiversity experiment. PLoS One 9:e93733. doi: 10.1371/journal.pone.0093733
Wang, D., Wu, G. L., Zhu, Y. J., and Shi, Z. H. (2014a). Grazing exclusion effects on above-and below-ground C and N pools of typical grassland on the Loess Plateau (China). Catena 123, 113–120. doi: 10.1016/j.catena.2014.07.018
Wang, D., Zhang, B., Zhu, L. L., Yang, Y. S., and Li, M. M. (2018). Soil and vegetation development along a 10-year restoration chronosequence in tailing dams in the Xiaoqinling gold region of Central China. Catena 167, 250–256. doi: 10.1016/j.catena.2018.05.007
Wang, K., Ma, J. Y., Li, M. Y., Qin, Y. S., Bao, X. C., Wang, C. C., et al. (2020). Mechanisms of Cd and Cu induced toxicity in human gastric epithelial cells: oxidative stress, cell cycle arrest and apoptosis. Sci. Total Environ. 756:149351. doi: 10.1016/j.scitotenv.2020.143951
Wang, R. Z., Yang, J. J., Liu, H. Y., Sardans, J., Zhang, Y. H., and Wang, X. B. (2022). Nitrogen enrichment buffers phosphorus limitation by mobilizing mineral-bound soil phosphorus in grasslands. Ecology 103:e3616. doi: 10.1002/ecy.3616
Wu, G., Kang, H. B., Zhang, X. Y., Shao, H. B., Chu, L. Y., and Ruan, C. J. (2010). A critical review on the bio removal of hazardous heavy metals from contaminated soils: issues, progress, eco-environmental concerns, and opportunities. J. Hazard Mater. 174, 1–8. doi: 10.1016/j.jhazmat.2009.09.113
Wu, Q. H., Leung, J. Y. S., Geng, X. H., Chen, S. J., Huang, X. X., Li, H. Y., et al. (2015). Heavy metal contamination of soil and water in the vicinity of an abandoned e-waste recycling site: implications for dissemination of heavy metals. Sci. Total Environ. 506, 217–225. doi: 10.1016/j.scitotenv.2014.10.121
Wu, Y. P., Li, Y. F., Zheng, C. Y., Zhang, Y. F., and Sun, Z. J. (2013). Organic amendment application influence soil organism abundance in saline alkali soil. Eur. J. Soil Biol. 54, 32–40. doi: 10.1016/j.ejsobi.2012.10.006
Xu, H., Detto, M., Fang, S. Q., Chazdon, R. L., Li, Y. D., Hau, B. C. H., et al. (2020). Soil nitrogen concentration mediates the relationship between leguminous trees and neighbor diversity in tropical forests. Commun. Bio. 3:317. doi: 10.1038/s42003-020-1041-y
Yan, K., Ranjitkar, S., Zhai, D. L., Li, Y. J., Xu, J. C., Li, B., et al. (2017). Current re-vegetation patterns and restoration issues in degraded geological phosphorus-rich mountain areas: a synthetic analysis of Central Yunnan, SW China. Plant Divers. 39, 140–148. doi: 10.1016/j.pld.2017.04.003
Yang, D., Zeng, D. H., Zhang, J., Li, L. J., and Mao, R. (2012). Chemical and microbial properties in contaminated soils around a magnesite mine in northeast China. Land Degrad. Dev. 23, 256–262. doi: 10.1002/ldr.1077
Yin, G. Z., Li, G. Z., Wei, Z. A., Wan, L., Shui, G. H., and Jing, X. F. (2011). Stability analysis of a copper tailings dam via laboratory model tests: a Chinese case study. Miner. Eng. 24, 122–130. doi: 10.1016/j.mineng.2010.10.014
Zeidler, M., Šipoš, J., Banaš, M., and Černohorský, J. (2021). The successive trend of vegetation confirms the removal of non-indigenous woody species as an insufficient restoration action. Biodivers. Conserv. 30, 699–717. doi: 10.1007/s10531-021-02113-x
Keywords: heavy metal, plant diversity, structural equation model, functional trait, complex biomass, functional diversity, nutrient element
Citation: Yuan X-q, Guo Z-l, Duan C-q, Yang J, Tang H, Li L-y, Li T and Liu C-e (2022) Alleviation of heavy metal stress and enhanced plant complex functional restoration in abandoned Pb–Zn mining areas by the nurse plant Coriaria nepalensis. Front. Ecol. Evol. 10:1006468. doi: 10.3389/fevo.2022.1006468
Received: 29 July 2022; Accepted: 26 September 2022;
Published: 10 October 2022.
Edited by:
Anna Gałązka, Institute of Soil Science and Plant Cultivation, PolandReviewed by:
Surendra Singh Bargali, Kumaun University, IndiaPurabi Saikia, Central University of Jharkhand, India
Copyright © 2022 Yuan, Guo, Duan, Yang, Tang, Li, Li and Liu. This is an open-access article distributed under the terms of the Creative Commons Attribution License (CC BY). The use, distribution or reproduction in other forums is permitted, provided the original author(s) and the copyright owner(s) are credited and that the original publication in this journal is cited, in accordance with accepted academic practice. No use, distribution or reproduction is permitted which does not comply with these terms.
*Correspondence: Chang-qun Duan, chqduan@ynu.edu.cn