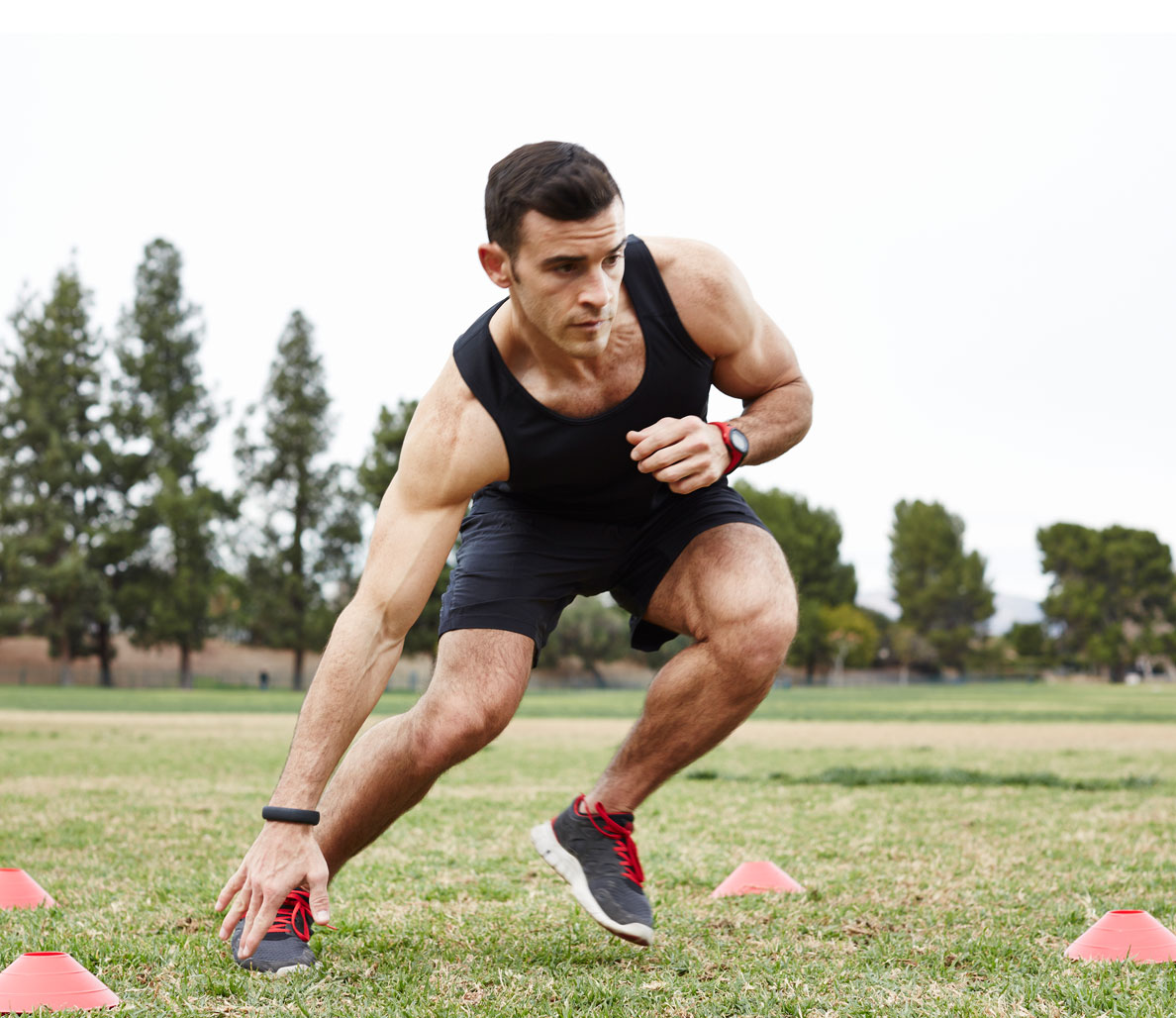
17 Oct Understanding the differences between speed, agility, and change of direction
Speed, agility, and change of direction are all components of field-based sports. Each one of these components are integral in performance of a team and each individual athlete. The development of these three components within the athletic population is a major driver of success and improved performance of the individual within their chosen sport of participation. Understanding the underlying biomechanics of speed, agility, and change of direction is important in developing the constructs to improve each of these entities within the athletic population.
Speed
Speed involves the process of accelerating and reaching maximal velocity (Haff & Triplett, 2016). Athletes incorporate speed in a linear component in track and field competitions whereas a multi-directional component exists in field orientated competitions such as soccer, football, lacrosse, and rugby. The underlying concept of speed as it pertains to the athletic population is sprinting. Sprinting is the process by which an athlete will reach maximal velocity and ideally in the shortest amount of time as possible (Mero, Komi, Gregor, 1992). An understanding of the underlying biomechanics of sprinting provide the constructs by which the strength and conditioning professional can develop programming to improve maximal velocity within the athletic population.
The underlying components of speed is the application of force allowing the athlete to accelerate, achieve a high velocity, and maintain a high velocity (Haff et al., 2016). Underlying this concept is the rate of force development (RFD). The rate of force development is defined as development of maximal force in minimal time (Haff et al., 2016). Maximum force in minimal time is equated to an index of explosive strength and power (Haff et al., 2016).
The process by which an athlete will create speed during the sprinting action requires the individual producing force overcoming gravity and creating a positive change in velocity (Haff et al., 2016). The development of force initially and in a rapid manner requires acceleration. Acceleration is defined as the rate of change of velocity (Young et al., 2015). Velocity and speed are often used interchangeably though for a proper discussion on speed, agility, and change of direction it is best to separate the terms (Haff et al., 2016). Speed is the rate at which an object covers distance whereas velocity has a vector quantity (Haff et al., 2016). Velocity describes both speed and direction (Haff et al., 2016).
Speed development via sprinting involves a rapid acceleration phase followed by a velocity maintenance phase (Coh et al., 2018). Initial acceleration and maintenance of velocity is contingent upon step frequency, average vertical force applied to the ground, and contact length (Mero et al., 1992). The biomechanical constructs underlying sprinting in the acceleration phase have the body tilted forward to direct ground reaction forces more horizontally (Mero et al., 1992). During this initial phase a shin angle of 45 degrees with a forward leaning torso will occur (Murphy et al., 2003). Arm swing plays a vital role in the acceleration phase and subsequent phases of sprinting in stabilizing the torso and creating vertical propulsion (Mero et al., 1992). Arm swing serves to counterbalance the rotational momentum created by the leg swing (Mero et al., 1992). After completion of the initial phase of acceleration as the body moves into velocity maintenance phase rate of acceleration will diminish as forward body lean lessens (Mero et al., 1992). The torso lean will become more upright as shin angle increases to 80-90 degrees (Mero et al., 1992). Once the maintenance phase of the steady state of velocity has been achieved with a relatively upright body position, a large amount of the power to maintain speed comes from the mechanical energy stored in the elastic elements of the stretching of the contractile muscles released after positive work (Mero et al., 1992). One component of this is the stretch-shortening cycle (Haff et al., 2016). After the maintenance phase is complete a deceleration will occur once completion of the intended distance is completed. The deceleration is the process of slowing the kinetic chain.
Agility and Change of Direction
Agility and change of direction as with sprinting involves the process of acceleration though these entities are comprised of additional components. Agility can be defined as a whole-body movement with change of velocity or direction in response to a stimulus (Young et al., 2015). Change of direction is defined as pre-planned and thus does not entail a reaction to a stimulus and subsequent change of direction and velocity (Young et al., 2015). It is important to recognize the variation between these two components even though overlapping of the underlying constructs and biomechanics are present. Agility and change of direction are closely connected and do share underlying biomechanical components in terms of acceleration, deceleration, body positions, and changes of direction. Though the cognitive and reactionary aspects of these two entities are different.
Sport specific situations in field and court sports are complex and dynamic in terms of movement. The kinetic chain is required to accelerate, decelerate, and execute patterning while reacting to stimuli and mentally processing predetermined patterning (Coh et al., 2018). Agility and change of direction have the common component of decision making (Coh et al., 2018). Decision making can be predetermined as in change of direction or reactive to stimuli in accordance to the definition of agility.
Outside of the cognitive abilities associated with agility and change of direction, the underlying biomechanical components of these entities have a connection to the concept of speed as well as an interdependent connection. Agility which comprises a rapid whole-body movement with a change of velocity or direction in a response to a stimulus has a connection to velocity (Young et al., 2015). The connection to velocity can be either an increase or decrease in speed. The increase in velocity is predicated upon acceleration of the kinetic chain. Acceleration as stated previously is the rate of change in velocity (Young et al., 2015). Underlying components of acceleration are rate of force development and impulse (Haff et al., 2016). The process of developing maximum force in a minimal amount of time and the product of force time curve are prevalent in agility as with speed development (Haff et al., 2016). Research on initial acceleration in field sports indicates the underlying components of stride frequency, stride length, foot contact, and foot flight time are defining in terms of the rate at which an athlete will accelerate in field sports (Murphy et al., 2003).
A second component associated with agility and change of direction is deceleration (Haff et al., 2016). Deceleration is not a prevalent component of in-line sprinting as these sports allow for the kinetic chain to slow down (decelerate) gradually. Whereas in court and field sports deceleration occurs very rapidly. Deceleration can be defined as a reduction in the rate of speed (Young et al., 2015). Deceleration in terms of agility and change of direction typically occurs at a very rapid rate with a change of direction and reacceleration to be occurring immediately thereafter.
Deceleration involves slowing the body’s center of mass via the process of applying as much force as possible over minimal time to allow a stop or movement in a different direction (Hewitt et al, 2011). Proper joint angles, leg kinematics, and muscle tension is imperative in deceleration and the resistance of forward momentum (Hewitt et al., 2011). A shortened gait cycle occurs in this process to absorb eccentric forces, ground contact of the landing leg occurs ahead of body’s center of mass differing this component from acceleration and ground contact occurs with dorsiflexion of the ankle and entire foot as the heel creates a braking action (Hewitt et al., 2011). These components allow for the rapid reduction in velocity to occur with the opportunity to re-accelerate. Additional components of the deceleration phase relative to linear acceleration is an erect or posterior lean of the upper body (Hewitt et al., 2011).
A final component associated with agility and change of direction is the transition from deceleration to re-acceleration and position of the trunk (Haff et al., 2016). Controlling the trunk in deceleration and reorientation of the trunk with a change in vector allows for a much more effective re-acceleration (Haff et al., 2016). During deceleration especially in a linear direction the body’s center of gravity will be posterior of the plant leg (Hewitt et al., 2011). After completion of deceleration, a reorientation of the body’s center of gravity and upper extremities will be required to accelerate with the appropriate body lean in the new intended direction (Haff et al., 2016). A low center of mass appears to assist in this process allowing for center of gravity to be directed effectively (Haff et al., 2016). Arm action in both deceleration, reorientation, and into reacceleration should be powerful to facilitate leg drive (Haff et al., 2016). It is also important to ensure the arm action is not counterproductive resulting in a decrease in efficiency of deceleration or reorientation of the body (Haff et al., 2016).
Sample Progression Cycles for the 5-10-5, lateral depth jumps, and hurdle hops drills
Progression is the process of continually challenging the kinetic chain via increases in intensity to elicit a training effect from the exercises or modalities executed by the athlete or individual (Haff et al., 2016). The 5-10-5, lateral depth jumps, and hurdle hops are three drills in which the inclusion of the principle of progression can be of great benefit. All three of the exercises/drills are very advanced and the process of progression will incorporate the exercises/drills to be utilized in progressing an athlete in the execution of the 5-10-5, lateral depth jumps, and hurdle hop exercises.
The hypothetical progressions will utilize a population of collegiate level athletes participating in field orientated sports. The underlying components of joint mobility, soft tissue flexibility, individual corrective exercise programming, strength, strength endurance, and power development are all aspects of these hypothetical athletes programming within their strength and conditioning programs. Though all the actual exercises for each of these physiological components may not be discussed directly in progressions derived for each of the drills/exercise
The 5-10-5 is an agility drill or test comprised of rapid acceleration, deceleration, and changes of direction. The athlete during the execution of this drill will accelerate from the starting line towards a cone set to their left at 5 yards, decelerate, and change direction to accelerate to a second cone positioned 5 yards laterally from the initial starting position. The athlete will complete the drill by decelerating at the second cone, changing direction, and accelerating through the starting/finish line of the drill.
Improving an athlete in this drill would require improvement in the areas of acceleration, deceleration and change of direction. One overarching component and physiological component to improve is the rate of force development. The development of maximum force in a minimal time is connected to the development of explosive strength (Haff et al., 2016). This physiological aspect would be address in the athlete’s strength and conditioning program with the inclusion of Olympic lifts at a high percentage of 1 RM. Outside of the Olympic lifting to address explosive strength. A series of drills would be implemented to address acceleration, deceleration, and change of direction. The progression would be set up in a systematic series of blocks where each block would progress the intensity or complexity of the drills. Each block would be 4 weeks in length, include drills to address acceleration, deceleration, and change of direction. Drills would be performed 2 times per week in the off-season on the non-lifting days of the athletes which in this scenario is Tuesday and Thursday. Prior to execution of block drills, the athlete performs a dynamic warm-up with the inclusion of a series of form running drills. The goal of the form running drills are two-fold in terms of preparing the kinetic chain for field drills to be executed in addition to improving sprinting technique for acceleration.
Block One
Drill | Emphasis | Volume |
Lean, Tall, & Fall | Initial Step Acceleration | 4 sets x 5 yards (30 sec.) |
Partner Resistant Sprints | RFD, Acceleration | 2 sets x 15 yards (1.5 min rest) |
Decelerations Forward | Deceleration, Change of Direction | 2 sets x 20 yards (30 sec.) |
Line-Stops | Lateral Deceleration, Change of Direction | 2 sets x 10 yards (30 sec.) |
Block Two
Drill | Emphasis | Volume |
Push Up Starts | Initial Step Acceleration | 4 sets x 5 yards (30 sec.) |
Sled Tows | RFD, Acceleration | 2 sets x 15 yards (1.5 min.) |
Decelerations Lateral | Lateral Deceleration, Change of Direction | 2 sets x 10 yards (30 sec.) |
Z-Drill | Change of Direction, Acceleration, Deceleration | 2 sets (30 sec.) |
Block Three
Drill | Emphasis | Volume |
Accelerators from Crouch | Acceleration | 3 sets x 15 yards (30 sec.) |
Assisted Sprints | Overspeed | 2 sets x 15 yards (1.5 min.) |
T-Test Drill | Acceleration, Deceleration, Change of Direction | 4 sets (30 sec.) |
The lateral depth jump is a very complex and high intensity plyometric drill. The progression to the lateral depth jump for the athlete will require a process of beginning with low intensity plyometric drills addressing landing mechanics and deceleration. The progression would continue with increasing plyometric intensity and introducing the lateral component of drills. A block type progression would be implemented with increases in intensity beginning with standing jumps, progressing to box drills, and completing with depth jumps (Chu et al., 2011). Each block would be 4 weeks in length and be contingent upon development and mastery by the athlete (Chu et al., 2011). Plyometrics would be performed 2 times per week and incorporated into the strength and conditioning programs of the athletes.
Block One: Split Squat Jump – 60 foot contacts/ 3 sets – 1:2work/rest ratio
Block Two: Lateral Jump Over Barrier – 60 foot contacts/3sets – 1:2 work/rest ratio
Block Three: Lateral Box Jump – 30 foot contacts/3 sets – 1:2 work/rest ratio
The hurdle hops exercise could be classified as a multiple jump linear plyometric exercise (Chu et al. 2016). The process be which the athlete would be progressed to this exercise would be very similar to the lateral depth jump. The process would begin with low intensity plyometric drills addressing landing mechanics and progress to consecutive linear jumps. A block progression would be implemented with standing jumps and progressing to low intensity multiple jumps (Chu et al., 2011). Each block would be 4 weeks in length and again contingent upon mastery by the athlete. Plyometrics would be performed 2 times per week and incorporated into the strength and conditioning program of the athletes.
Block One: Two Foot Ankle Hop – 60 foot contacts/3 sets – 1:2 work/rest ratio
Block Two: Standing Linear Jump Over Barrier – 60 foot contacts/3 sets – 1:2 work/rest ratio
Block Three: Front Consecutive Cone Hops – 30 foot contact/3 sets – 1:2 work/rest ratio
Resources
Boyle, M. (2014) Functional training for sports 2nd edition. Champaign, IL: Human Kinetics.
Cetin, E. Hindistan, E. Ozkaya, Y. (2017) Effect of different training methods on stride parameters in speed maintenance phase of 100-m sprint running. Journal of strength and conditioning research, 32 (5) 1263 – 1272.
Coh, M. Vodicar, J. Zvan, M. Simenko, J. Stodolka, J. Rauter, S. Mackala, K. (2018) Are change-of-direction speed and reactive agility independent skills even when using the same movement pattern? Journal of strength and conditioning research, 32 (7) 1929 – 1936.
Chu, D. (2011) Jumping into plyometrics 2nd edition. Champaign, IL: Human Kinetics.
Clark, M. Lucent, S. (2010) NASM essentials of sports performance training. Baltimore, MD: Lippincott, Williams, & Wilkins.
Haff, G. Triplett, T. (2016) Essentials of strength training and conditioning 4th edition. Champaign, IL: Human Kinetics.
Hewitt, J. Cronin, J. Button, C. Hume, P. (2011) Understanding deceleration in sport. Strength and conditioning journal, 33 (1) 47-52.
Mero, A. Komi, P. Gregor, R. (1992) Biomechanics of spring running. Journal of sports medicine, 13 (6) 376 – 392.
Murphy, A. Lockie, R. Couts, A. (2003) Kinematic determinants of early acceleration in field sport athletes. Journal of sports science medicine, 2 (4) 144 – 150.
Young, W. (2015) Agility and change of direction speed are independent skills: Implications for agility invasion in sport. International journal of sports science and coaching, 10 (1) 159 – 167.